Solid lipid nanoparticles for biologics and drug encapsulation
This highlighted paper, published in Drug Delivery and Translational Research (2023), is a joint research project between Queen's University Belfast, Immunocore Ltd., and the University of Bari.
It presents a microfluidic approach, integrating Fluigent's Flow EZ, for producing solid lipid nanoparticles (SLNs) capable of encapsulating biologics and other active ingredients. This approach achieved small particle sizes (160-320 nm) and high encapsulation efficiencies (47-90%) for hydrophilic and lipophilic compounds. In vitro results suggested sustained drug release profiles, making this method a significant advancement in SLN production, especially for challenging molecules.
A Queen’s University Belfast Paper
Paper: Weaver, E.; Sommonte, F.; Hooker, A.; Denora, N.; Uddin, S.; Lamprou, D. A. Microfluidic Encapsulation of Enzymes and Steroids within Solid Lipid Nanoparticles. Drug Deliv. and Transl. Res. 2023. https://doi.org/10.1007/s13346-023-01398-5.
The Lamprou lab, affiliated with Queen’s University Belfast, specializes in three main areas: nanoparticles for imaging and therapy, lab-on-a-chip technology, and therapeutic implants. Their interdisciplinary approach has driven innovation in healthcare since 2012, developing emerging technologies and novel drug delivery devices. The lab is led by Professor Dimitrios Lamprou, a leading expert in pharmaceutical technologies known for his significant contributions to 3D printing, microfluidics, and nanofibers, with over 150 peer-reviewed publications.The Lamprou lab, in collaboration with the Department of Pharmacy‑Pharmaceutical Sciences (University of Bari Aldo Moro) and Immunocore Ltd., used microfluidics to create eco-friendly lipid-based nanocarriers for encapsulating challenging active pharmaceutical ingredients (APIs).
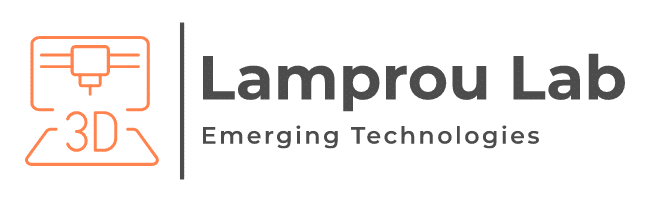
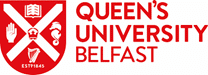
What are the challenges for biologics and drug delivery?
Biologics are complex molecules derived from living sources, including mRNA vaccines like those used against COVID-19.1,2 Protecting biological drugs from the challenges posed by various inhospitable internal conditions in the body, such as proteases and pH variations, remains a difficult challenge. Since biologics are expensive to produce, optimizing the formulation process is crucial for mass production. There is therefore a growing interest in oral delivery to promote better patient compliance. One promising method is nanoformulation, specifically using solid lipid nanoparticles as a barrier for the active pharmaceutical ingredient post-administration. 3
What makes Solid Lipid Nanoparticles effective for various drug types?
Solid Lipid Nanoparticles have proven their effectiveness in delivering various types of drugs, including chemotherapy drugs, genetic material, and anti-inflammatory medications, through their stability, targeting capabilities, and size.4
SLNs present a special structure with a hydrophobic core and a hydrophilic external layer, enabling them to encapsulate both hydrophilic and hydrophobic substances. The solid core, consisting of solid lipids like waxes and glycerides, is responsible for containing the drugs, particularly hydrophobic ones. On the other hand, the surfactant layer on the outside of an SLN enhances stability and targeted drug delivery and can also contain hydrophilic drugs. The choice of drug significantly influences the components used in lipid-based nanocarrier formulation, with cationic lipid cores and surfactants being important for mRNA and DNA delivery.5
Lipid-based nanoparticles are not limited to SLNs alone, but also include liposomes, niosomes, and exosomes, each with their own distinct properties, as summarized in Table 1.
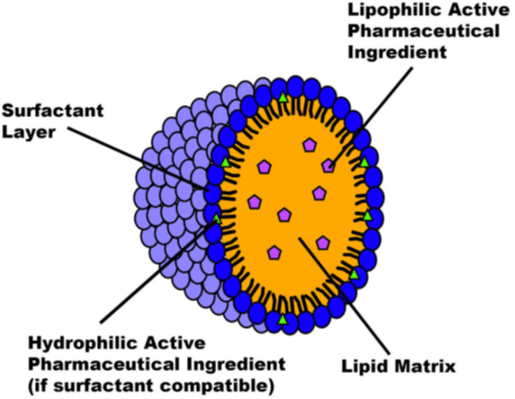
Figure 1: Structure of an SLN capable of encapsulating an API
Nanoformulation type | Formulation materials | Capable of encapsulating | Advantages | Disadvantages |
Solid Lipid Nanoparticles | • Waxes • Sterols • Surfactants | • Hydrophobic and hydrophilic | • Highly modifiable • High biocompatibility, including non-toxic degradation | • Require a cooling process for solidification • API leakage during storage • Complications caused by crystallisation |
Liposomes | • Phospholipids • Cholesterol | • Hydrophobic and hydrophilic | • Highly modifiable • Used for theranostic purposes • Simple synthesis | • Low skin permeability • Infrequently possess low mechanical strength |
Niosomes | • Non-ionic surfactants • Cholesterol | • Hydrophobic and hydrophilic | • Biocompatible and nonimmunogenic • Improve drug permeation through the skin • Less stringent storage requirements compared to liposomes | • Time-consuming to create • API leakage |
Exosomes | • Lipids • Proteins • Glycoconjugates | • Hydrophobic and hydrophilic • Genetic material | • Used for theranostic purposes | • Complex and expensive to artificially manufacture |
Table 1: Common lipid-based nanoformulations
How does microfluidics enhance SLN production for drug formulation?
Traditional bulk production methods for solid lipid nanoparticles, such as homogenization and microemulsification, have multiple drawbacks including unpredictable particle characteristics, low reproducibility and repeatability, and the environmentally harmful use of solvents.
To address these issues, microfluidics has emerged as a promising approach for lipid-based nanocarrier formulation and encapsulation of drugs.
Microfluidics has gained popularity in drug delivery due to its precise control of flow rates, device design, and mixing angles within submicron channels. These characteristics enable control over particle size, morphology, and encapsulation efficiency, as flow rates have a significant impact on these parameters. The microfluidic approach also improves time efficiency, allowing for continuous production within minutes, unlike traditional batch processes. As a result, microfluidics is particularly well-suited to formulations relying on self-assembly, like liposomes and SLNs, and has been employed for a wide range of APIs, including those used for gene therapy and addressing the COVID-19 pandemic.6,7,8
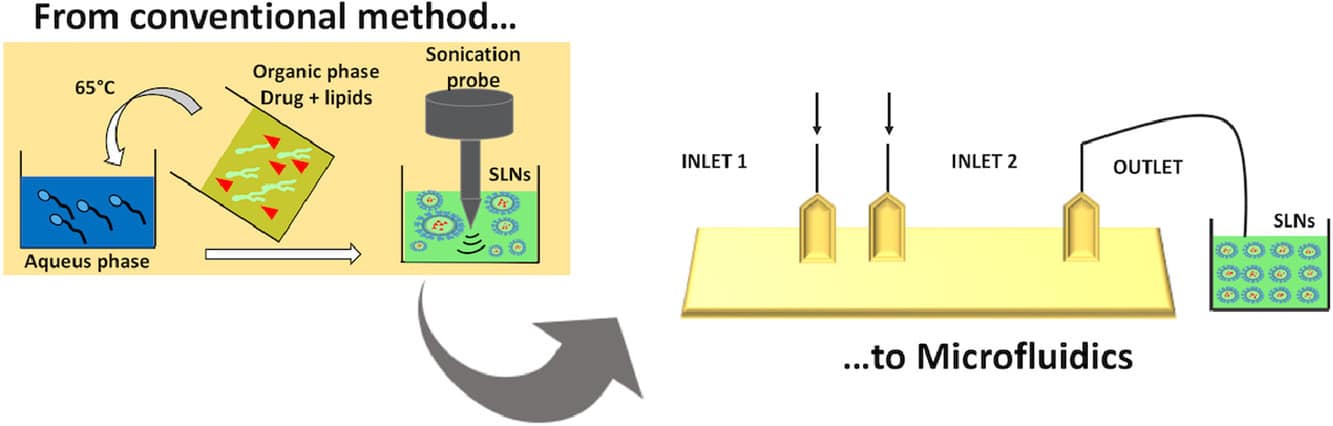
Figure 3 : Microfluidics, a promising approach for SLN nanoformulation.[9]
Aim of the study
To further explore this area of drug encapsulation, Edward Weaver et al. from the Lamprou Lab aimed to demonstrate microfluidics’ position at the forefront of solid lipid nanoparticle production in general, and for biologic SLNs specifically.
This paper examines the compatibility of trypsin (TRP) and testosterone (TES) for encapsulation in solid lipid nanoparticles using a microfluidic process integrating Fluigent’s Flow EZ. Testosterone was chosen as a positive non-biologic lipophilic API for comparison with previous bulk encapsulation research, while trypsin was evaluated for encapsulating a hydrophilic API in lipid-based nanocarriers. A combination of SLN materials, including tripalmitin (Tri-P), soybean lecithin (LEC), Tween 80 (T80), and cetyl palmitate (CP) in conjunction with Pluronic F68 (P68), was used for nanoformulation using the same experimental setup.
Solid Lipid Nanoparticle Production Method
Fluigent’s Flow EZ was used to synthesize various nanoformulations of solid lipid nanoparticles. The microfluidic device used was a Y-shaped inlet with etched herringbone channels. The overall system was maintained at a temperature of 60°C to ensure complete dissolution of the materials.
Eight different SLN formulations (F1-F8) were created, with materials dissolved at 60°C to match their final concentration (Table 2). For SLNs encapsulating APIs, TRP and TES were used at varying concentrations, with TRP in the aqueous phase and TES in the organic phase. The optimal flow rate ratio was determined to be 5:1 (aqueous to organic), and samples were collected. The ethanol excess was evaporated through vortex stirring, and SLN formulations were then refrigerated at 5°C for 24 hours.
The API-loaded SLNs were characterized by dynamic light scattering (DLS), zeta potential, atomic force microscopy (AFM), differential scanning calorimetry (DSC) and Fourier transform infrared spectroscopy (FTIR).
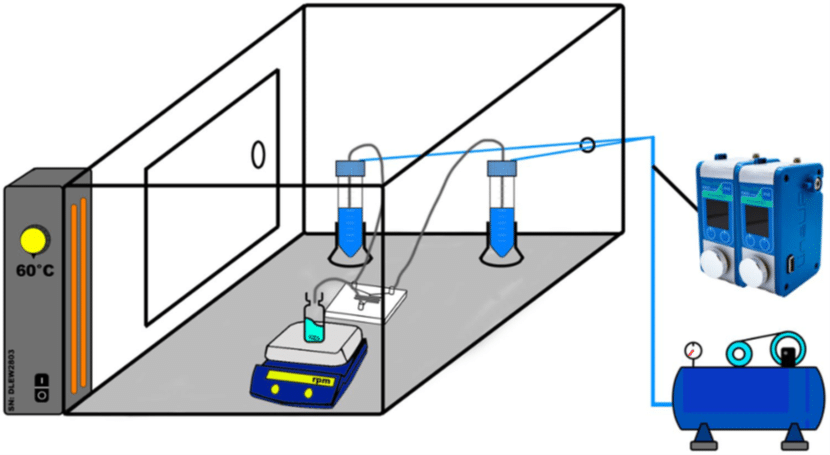
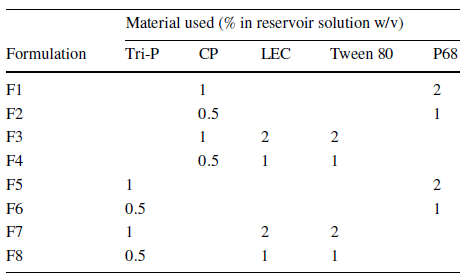
Partial results: Stable and monodisperse SLNs for high encapsulation efficiency.
Solid lipid nanoparticles produced from various combinations and concentrations (F1-F8) were first evaluated by DLS. When comparing non-loaded SLNs to the ones with API (trypsin or testosterone), a slight increase in particle size upon encapsulation was observed. Formulations F2 (CP, P68) and F8 (Tri-P, LEC, Tween 80) presented the most favorable particle sizes (150 -180 nm) and were thus selected as model formulations for the study. The choice of API has a lesser impact on particle size compared to the choice of materials. In fact, the main factor affecting particle size appeared to be the interaction between the waxy core material and the surfactant layer.
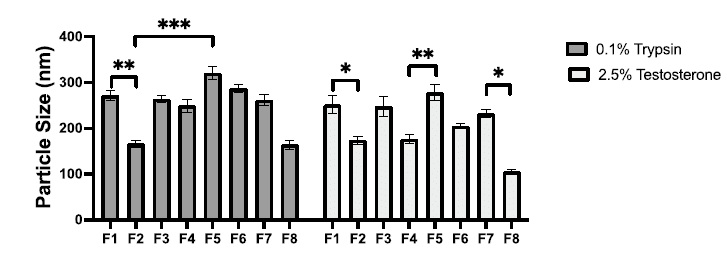
Figure 6 : Particle size measurements for formulations F1–F8.
“However, it was also found in the current study that when using a commercially available chip and the Fluigent system, halving the required concentrations provided more opportune particle diameter. This factor indicates the importance of considering both the system and the microfluidic environment that is being used for formulation. ”
AFM results aligned with DLS analysis, confirming the size range, although slightly enlarged due to drying. It also verified the uniform dispersion of SLNs that was achieved, suggesting great potential for future development.
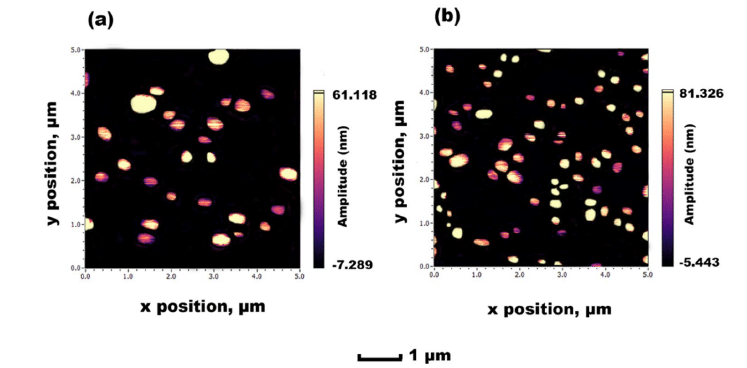
Figure 7: AFM images obtained for F8 SLN encapsulation: (a) TRP and (b) TES
Regarding stability studies, all of the formulations (F1-F8) appeared to function effectively. In particular, F2 and F8 demonstrated favorable stability characteristics, ensuring consistent and prolonged release.
FTIR spectroscopy confirmed the presence of TRP and TES in SLN formulations. Specific peaks in the spectra indicated the presence of these compounds in the formulations, thus demonstrating their encapsulation within the solid lipid nanoparticles.
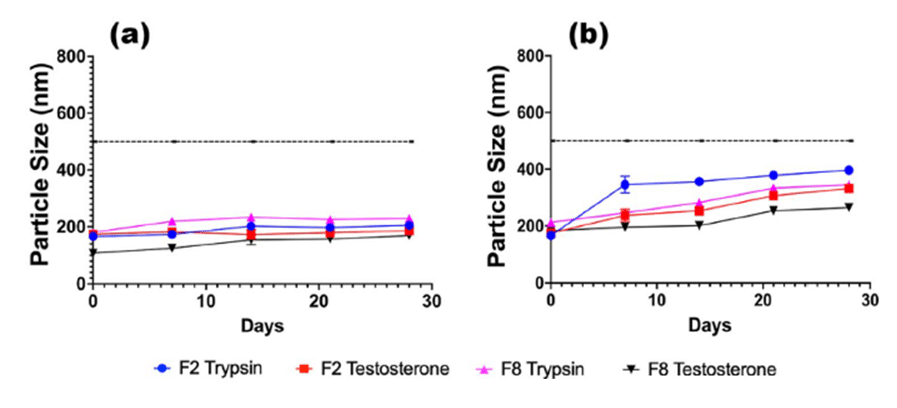
Figure 8: Stability for TRP and TES-loaded F2 and F8 formulations, stored at a) 5 °C and b) 37 °C
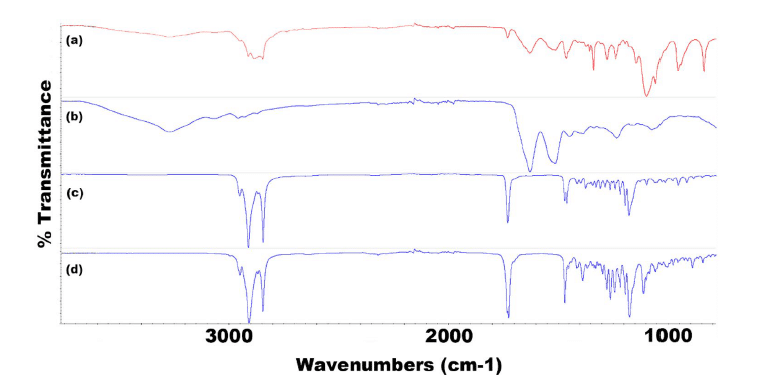
Figure 9: FTIR spectra for all components of F2 TRP showing (a) F2 TRP-encapsulated SLNs, (b) TRP, (c) CP, and (d) P68
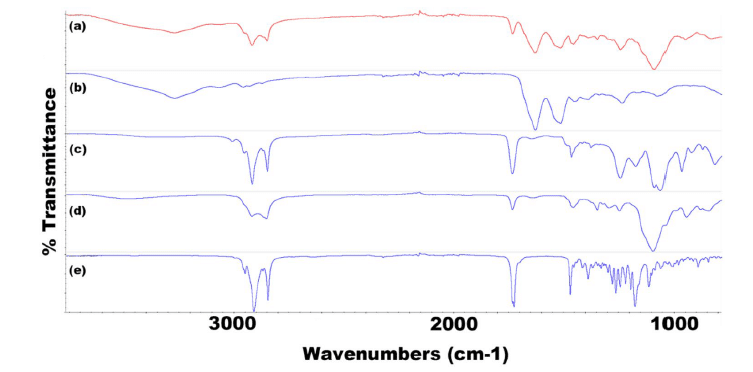
Figure 10: FTIR spectra for all components of F8 TRP showing (a) F8 TRP-encapsulated SLNs, (b) TRP, (c) LEC, (d) T80, and (e) Tri-P
Finally, the encapsulation efficiency (EE) and drug release with F2 and F8 formulation were evaluated. For testosterone, the encapsulation efficiency was high for both nanoformulations, showing a slight improvement over traditionally used encapsulation methods such as emulsification and homogenisation.10,11 Moreover, the release of TES from SLNs was around 65% for F2 and 45% for F8 within 72 hours, in agreement with the therapeutic range.
Regarding the encapsulation of TRP within solid lipid nanoparticles, the EE with the F2 formulation was 47% and showed controlled trypsin release, making it a suitable lead formulation compared to F8 (EE: 7%). This result is promising for future enhancement, taking into consideration that this encapsulation had not been attempted before with microfluidics.
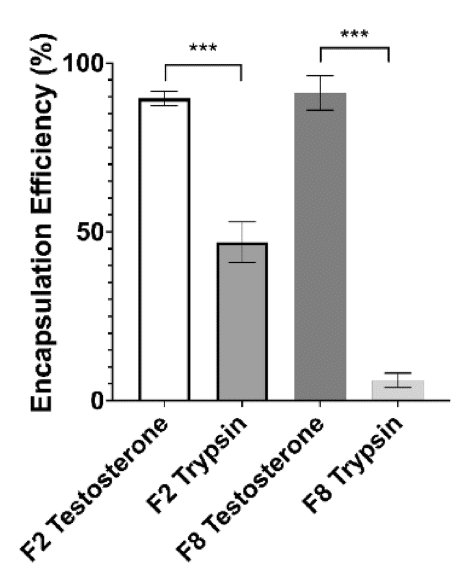
Figure 11: Encapsulation efficiency for F2 and F8 formulations encapsulating both TRP and TES
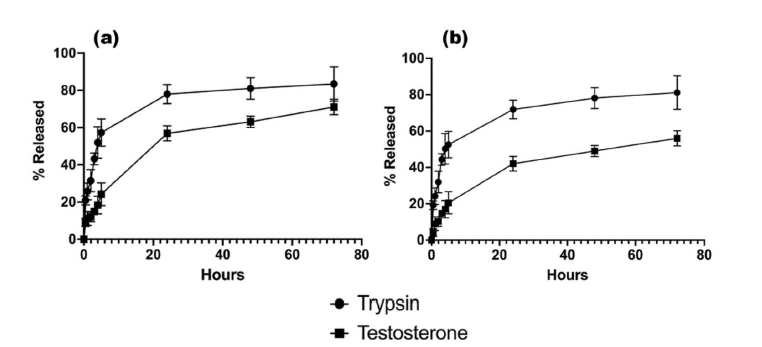
Figure 12: Drug release displayed as % total release from encapsulated active pharmaceutical ingredient for (a) F2 and (b) F8
Conclusion
In this paper highlight, E. Weaver et al. from the Lamprou lab developed a microfluidic approach for solid lipid nanoparticle production and API encapsulation. Fluigent’s Flow EZ was used to provide controlled and constant flow rates, contributing to the creation of small homogeneous SLNs. Selecting appropriate SLN materials was essential to matching specific APIs due to their varying encapsulation capabilities. The nanoformulation which included low-concentration CP and P68 was shown to be the most promising.12 This microfluidic method was both reproducible and eco-friendly, making it possible to encapsulate previously challenging molecules.
Related products
Related Content
Microfluidics for Pharmaceutical Applications
Read moreWebinar – Microfluidics in the manufacturing of sustainable nanomedicines
Read more
Expertises & Resources
-
Microfluidic Application Notes 1-10 microns PLGA microsphere production using the RayDrop Read more
-
Microfluidics Article Reviews A mRNA encapsulation platform integrating Fluigent’s FlowEZ Read more
-
Microfluidics Article Reviews Microfluidic technology for engineered nanoparticles in nanomedicine Read more
-
Microfluidic Application Notes PLGA microcapsules synthesis Read more
-
Expert Reviews: Basics of Microfluidics Microfluidics for vaccine development Read more
-
Microfluidic Application Notes Liposome Nanoparticle Synthesis Read more
-
Expert Reviews: Basics of Microfluidics Flow control for droplet generation using syringe pumps and pressure-based flow controllers Read more
References
- (1) Anselmo AC, Gokarn Y, Mitragotri S. Non-invasive delivery strategies for biologics. Nat Rev Drug Discovery. 2019;18(1):19–40.
- (2) Schoenmaker L, et al. mRNA-lipid nanoparticle COVID-19 vaccines: structure and stability. Int Pharm. 2021;601: 120586 .
- (3) Mishra V, et al. Solid lipid nanoparticles: emerging colloidal nano drug delivery systems. Pharmaceutics. 2018;10(4):191.
- (4) Mura P, et al. Evaluation and comparison of solid lipid nanoparticles (SLNs) and nanostructured lipid carriers (NLCs) as vectors to develop hydrochlorothiazide effective and safe pediatric oral liquid formulations. Pharmaceutics. 2021;13(4):437.
- (5) Sommonte F, et al. The complexity of the blood-brain barrier and the concept of age-related brain targeting: challenges and potential of novel solid lipid-based formulations. J Pharm Sci. 2022;111(3):577–92.
- (6) Jaradat E, et al. Microfluidic paclitaxel-loaded lipid nanoparticle formulations for chemotherapy. Int J Pharm. 2022;628:122320.
- (7) Jain V, et al. Microfluidic device based molecular self-assembly structures. J Mol Liq. 2022: 119760.
- (8) Roces CB, et al. Manufacturing considerations for the development of lipid nanoparticles using microfluidics. Pharmaceutics. 2020;12(11):1095
- (9) Arduino I, et al. Preparation of cetyl palmitate-based PEGylated solid lipid nanoparticles by microfluidic technique. Acta Biomater. 2021;121:566–78.
- (10) Tajbakhsh M, et al. An investigation on parameters affecting the optimization of testosterone enanthate loaded solid nanoparticles for enhanced transdermal delivery. Colloids Surf A. 2020;589:124437.
- (11) Doktorovova S, Souto EB, Silva AM. Hansen solubility parameters (HSP) for prescreening formulation of solid lipid nanoparticles (SLN): in vitro testing of curcumin-loaded SLN in MCF-7 and BT-474 cell lines. Pharm Dev Technol. 2018;23(1):96–105.
- (12) Weaver, E.; Sommonte, F.; Hooker, A.; Denora, N.; Uddin, S.; Lamprou, D. A. Microfluidic Encapsulation of Enzymes and Steroids within Solid Lipid Nanoparticles. Drug Deliv. and Transl. Res. 2023.