The Raydrop | A new droplet generation device based on non-embedded co-flow-focusing
Droplet microfluidics has emerged as a powerful tool for various applications ranging from fundamental research to single-cell analysis. However, the design of droplet generation chips has been limited by the planar flow-focusing configuration implemented in polymer or glass chips. In contrast, glass capillary-based droplet production devices have shown improved performance, but have been difficult to implement due to capillary centering and poor flexibility for droplet production.
To overcome these limitations, Fluigent has developed a new system called Raydrop, which is a non-embedded co-flow-focusing microfluidic droplet generator.
A new configuration to fill the gap in the design of microfluidic droplet generators
In recent years, droplet microfluidics has become an important tool for many different applications, including fundamental studies on emulsification, crystallization, chemical reaction, molecular encapsulation, particle synthesis, digital PCR, and single-cell analysis.
Design of the droplet generation chip
Most commercial microfluidic droplet generators rely on the planar flow‑focusing configuration implemented in polymer or glass chips. This geometry has many limitations, such as the need for specific coatings, or the use of dedicated surfactants. In contrast, glass capillary‑based droplet production devices are a great improvement, as the dispersed phase is never in contact with the walls of the outer capillary (Figure 1a,b).
They have been difficult to implement (centering of the capillary), and commercially available designs have shown poor flexibility for droplet production (> 100 µm diameter, < 1 kHz generation rate). Centering can be simplified by inserting two circular capillaries into a square outer flow capillary (Figure 1c,d), but the related manufacturing methods limit large-scale production, and high-throughput capillary-based droplet production has still not been achieved. A new configuration offers a promising alternative by placing the extraction tube in front of the injection tube without any surrounding confinement (see Fig. 1e). However, this system works only in the jetting regime, which does not guarantee the droplet monodispersity associated with the dripping regime.
Raydrop: A Non-Embedded Co-Flow-Focusing Microfluidic Droplet Generator
The authors present the Raydrop: a new system based on the latter configuration, but in which the dripping regime is enforced by using an injection capillary of smaller diameter than the extraction capillary (Figure 1f). This is achieved with an improved combination of cutting-edge machining and 3D printing techniques. This non-embedded design presents the characteristics of both a co-flow and a flow-focusing configuration, and is described as a “non-embedded co-flow-focusing” design. This configuration fills the gap in the design array of microfluidic droplet generators.
The Raydrop is an easy-to-use, commercially available microfluidic chip which makes it possible to generate droplets with outstanding monodispersity.
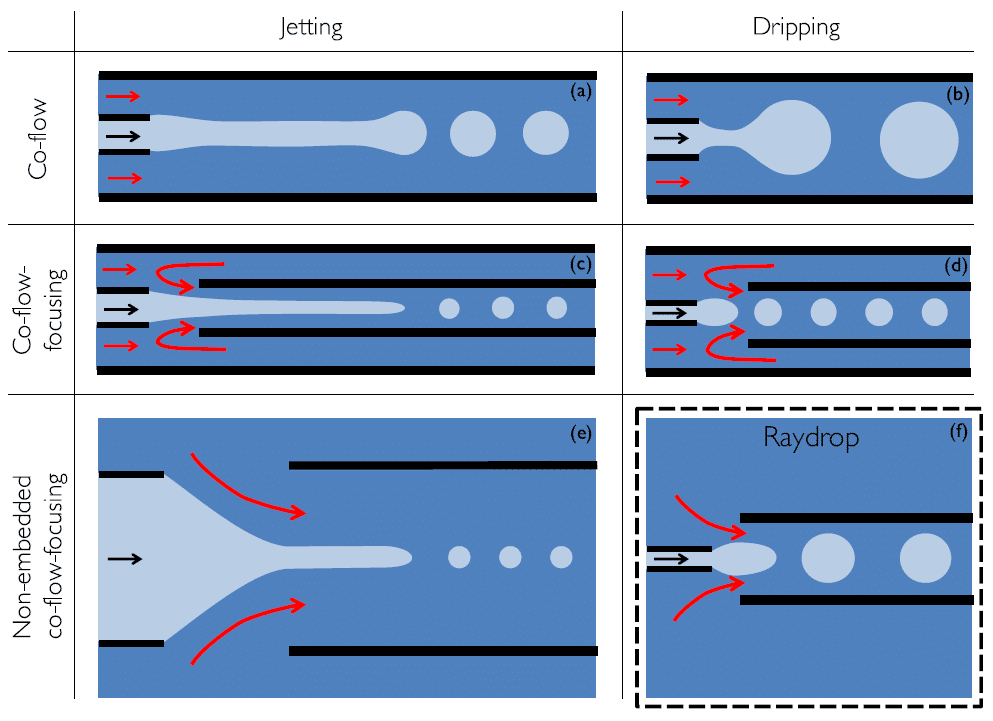
The Raydrop: a device based on the alignment of two capillaries immersed in a pressurized chamber
The Raydrop consists of a metallic pressurized chamber filled with a continuous phase. Two inserts supporting the glass capillaries (injection and extraction capillaries) are introduced on the lateral sides so that the capillaries are perfectly aligned and almost in contact at the center of the chamber (see Figure 2a). The dispersed phase flows from the injection capillary and meets the continuous phase at the intersection to the extraction capillary (see Figure 2), which results in the capillary-based technique for droplet production. Droplets are generated and flow through the extraction capillary. The 3D-printed micro-nozzle connected at the tip of the injection capillary (see figure 2 d) enforces the dripping regime of droplet formation.
The capillaries are held in the inserts so that alignment is guaranteed for all combinations, and a fixed gap between the nozzle and the extraction capillary is maintained, ensuring the proper production of droplets in the dripping regime. Two glass windows on the top and bottom faces of the device seal the chamber and allow for observation of the droplets.
The technological breakthrough of this design is two-fold:
- It enables high-throughput generation of monodisperse droplets, intrinsic to the dripping regime, for a wide variety of fluids.
- It benefits from specific fabrication techniques and materials compatible with large-scale production of the device.
Additionally, the device is plug-and-play thanks to the standard connections and the ability to easily assemble and disassemble all parts for cleaning.
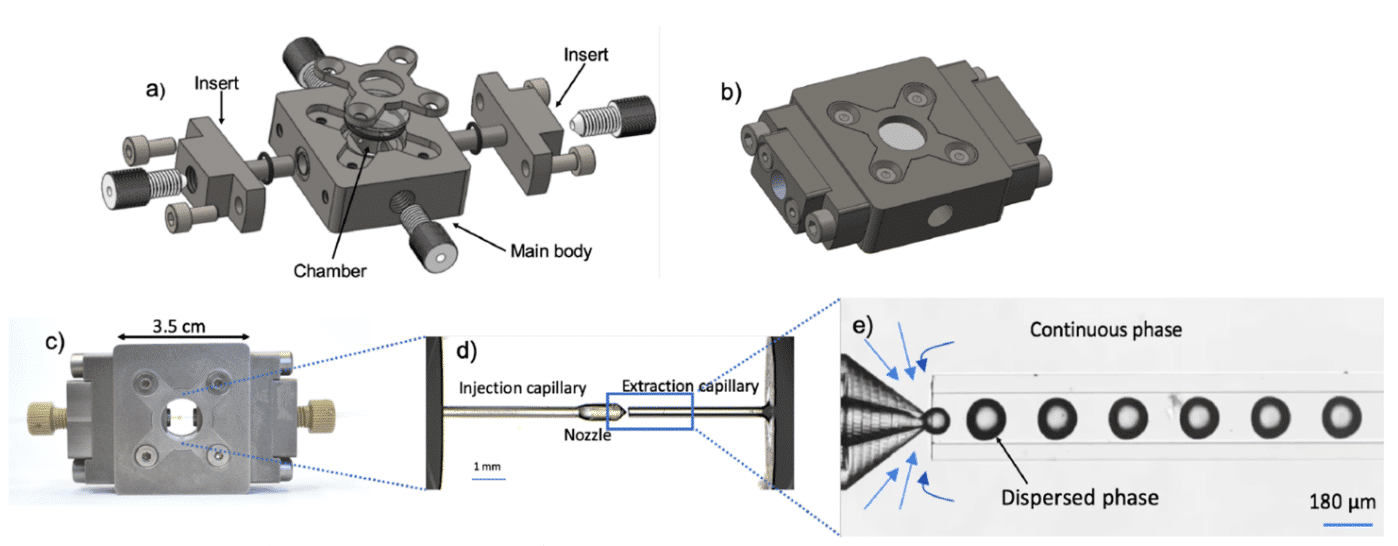
Figure 2: (a) Exploded view and (b) assembled view of the Raydrop. (c) The Raydrop with the injection and extraction glass capillaries. (d) Zoom through the top window of the two capillaries aligned in the chamber filled with the continuous phase. (e) Zoom on the capillary-based droplet generation area.
Generating droplets inside a microfluidic capillary using the Raydrop
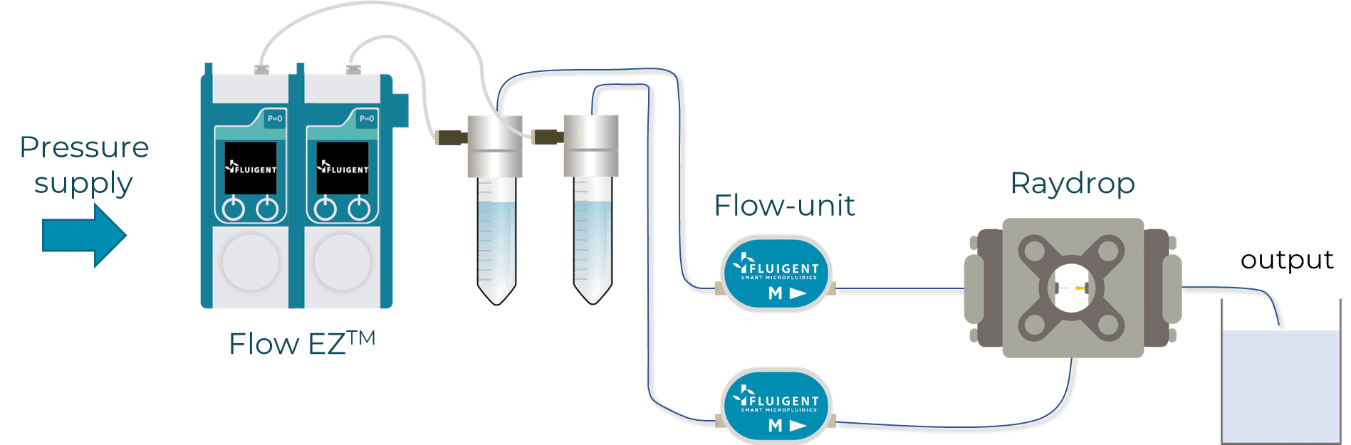
Figure 3: Setup used for droplet production using the Raydrop. The flows are controlled using a pressure flow controller. The flow rates are measured using flow-meters.
Setup description for capillary-based droplet generation
Using the Fluigent Flow-EZ pressure pumps, fluids are injected into the inner capillary (dispersed phase) and the chamber (continuous phase). Flow rates are measured using Flow Units. Different glass capillary geometries can be used and interchanged depending on the required droplet size and frequency. These geometries are referred to as “couples”. Here, two couples were used. Couple 1 consists of a nozzle of 30 µm diameter and a 150 µm diameter extraction capillary, while couple 2 uses a nozzle of 60 µm diameter and a 300 µm diameter extraction capillary. Qc and Qd are respectively defined as the flow rate of the continuous and dispersed phase.
Figure 4 shows water in mineral oil emulsions with droplet diameter and frequencies reached when using two different couples. The jetting-dripping regimes are also displayed. Using couple 1 (figure 4a), droplets with radius R ranging from 50 to 120 µm are produced, with frequencies ranging from 500 to 5000 Hz, at high monodispersity: the coefficient of variation (CV) is lower than 1%. Also, the dripping-jetting transition reaches a plateau for Qc = 200 μL/min (figure 4a), indicating that the dripping-jetting transition is only determined by the geometry in this region.
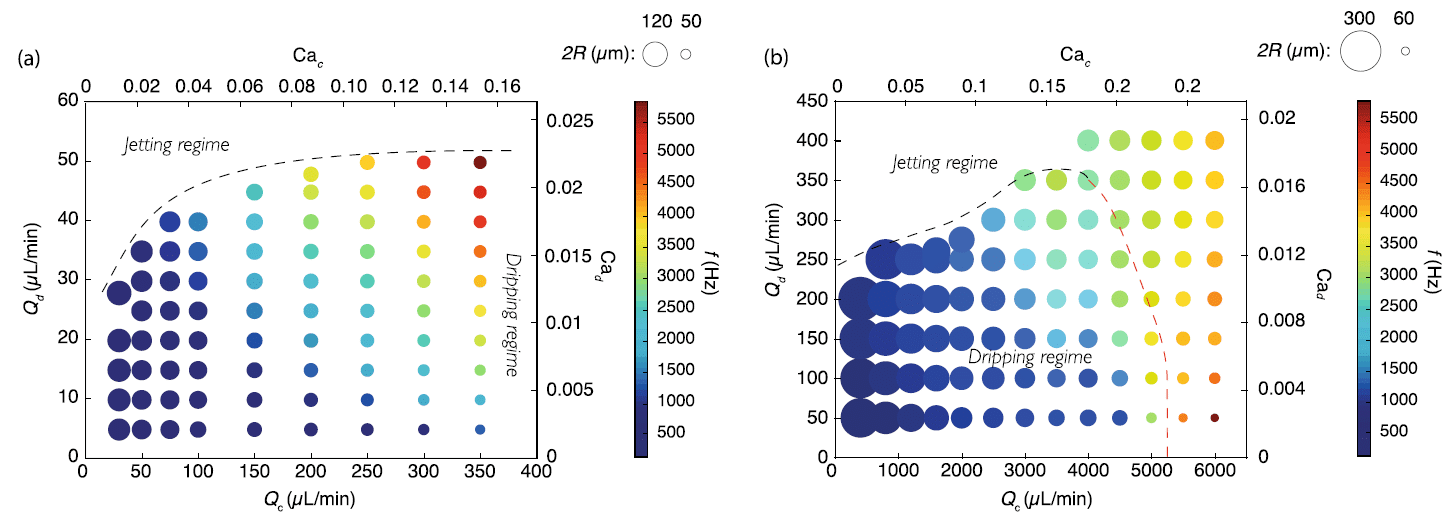
Figure 4: Size and production frequency of water droplets in mineral oil as a function of the continuous and dispersed flow rates Qc and Qd, respectively, with (a) couple 1 and (b) couple 2 geometries.
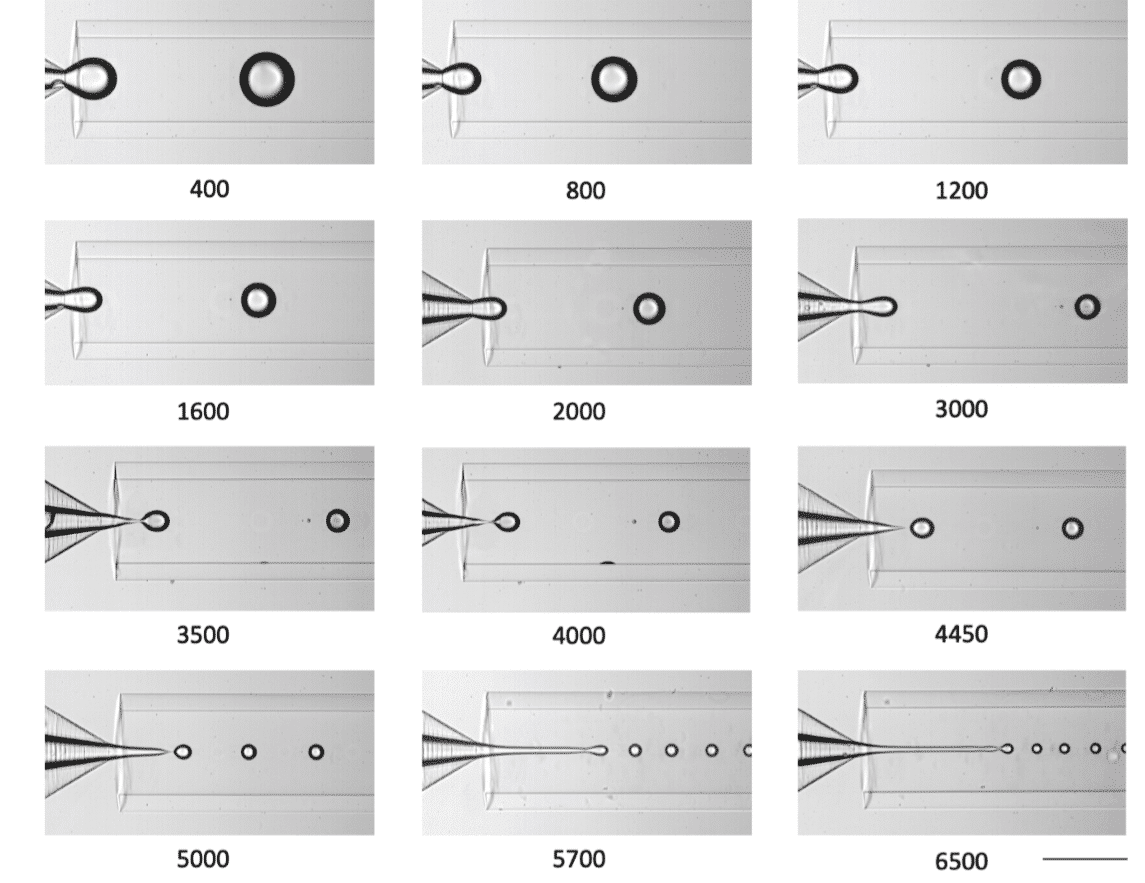
Figure 5: Water droplets in mineral oil using couple 2. Qd is fixed at 50 μL/min while the values of Qc are reported below each image (μL/min). The scale bar is 450 μm.
Using couple 2, capillary-based droplet production is possible with droplets having a radius R ranging from 60 to 300 µm are generated, with frequencies ranging from 500 to 5 000 Hz. Two dripping-jetting transitions can be observed, as this couple allows us to reach higher flow rate values. A transition is observed when increasing Qd (black dashed line in Fig. 4b), and another transition when Qc is increased above a threshold value (red dashed line in Fig. 4b).
Interestingly, this second transition at high Qc separates a monodisperse regime with CV < 1% from a polydisperse regime with CV > 4% (a value still much smaller than typical CV values observed in the jetting regime, usually larger than 10%). This transition is illustrated in Figure 5, where Qd is kept constant while Qc is increased. Note that no polydisperse regime seems to be reached whatever the value of Qc.
A theoretical model to predict droplet size and generation regime
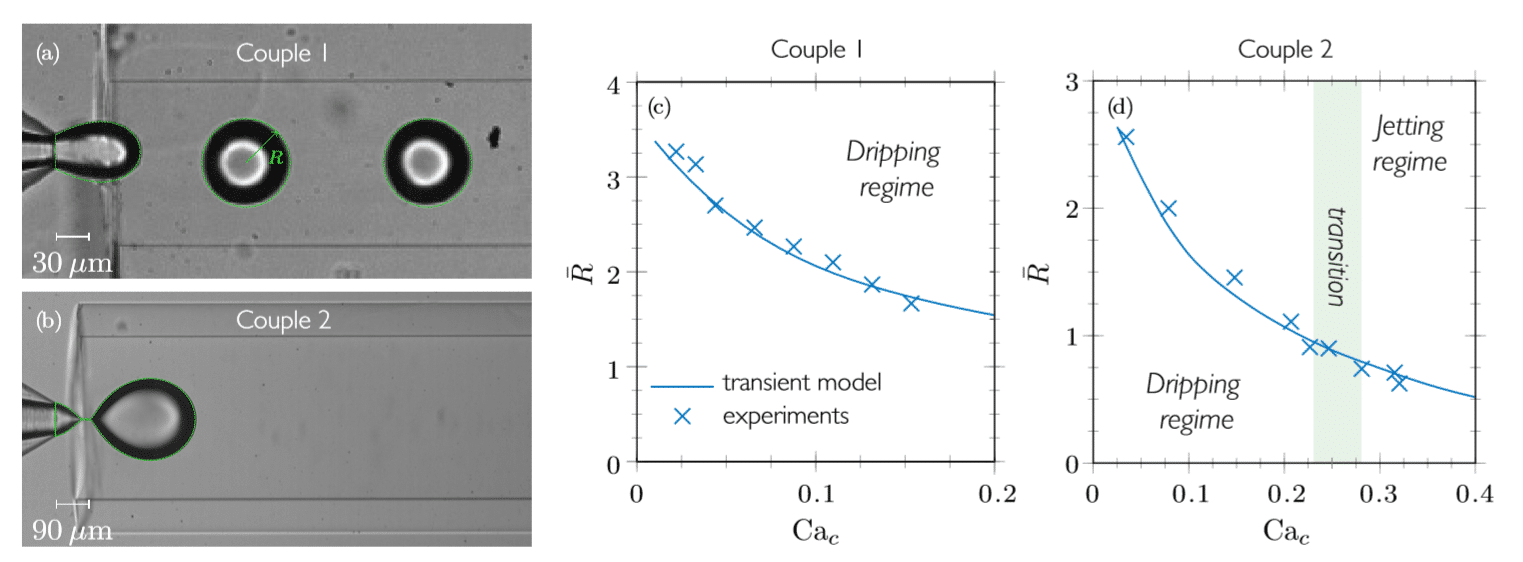
Figure 6: Comparison between transient simulations (green lines) and experimental pictures for the formation of water drops in mineral oil.
The authors make use of continuum and fluid mechanics to develop a highly sophisticated model of capillary-based droplet production. The details can be found in the paper. This system of equations is solved using the finite element method (FEM). This model is first validated by comparing with experimental data, as shown in figure 6 (where Cac is the capillary number of the continuous phase). It is then used to study the effect of several parameters such as fluid viscosity, inertia, and geometrical parameters (nozzle inclination angle, nozzle-capillary distance …) on droplet formation.
Using this model and quasi-static simulations (Qd << Qc) it is possible to predict droplet size as a function of several geometrical parameters. Figure 7 shows the effect of (a) the extraction capillary radius, (b) the nozzle-capillary distance and (c) the nozzle inclination on droplet size. This model is thus a great tool for end users, as it allows them to find an appropriate set of parameters for a targeted droplet size and generation regime.
To make it easier for end-users to determine the set of parameters needed to achieve the desired droplet size with the Raydrop, we developed a calculator.
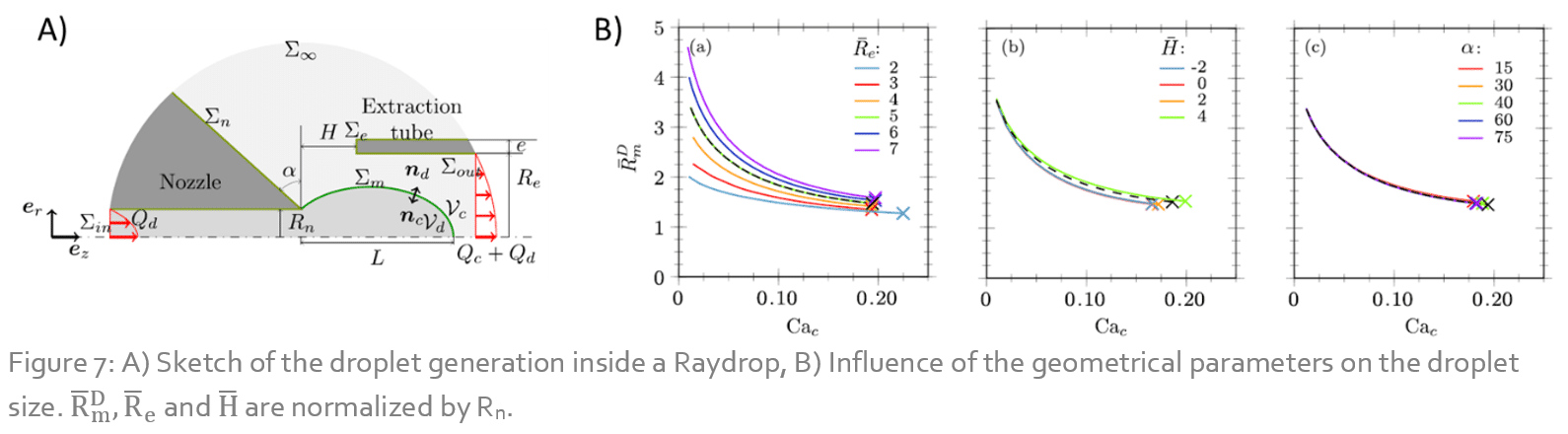
Raydrop versatility in generating capillary-based droplets
Using the Raydrop, it is also possible to generate bubbles and droplets involving a wide variety of fluid pairs. This universality is demonstrated in Figure 8, where the Raydrop was used to generate air-in-oil, ethanol-in-oil, water-in-oil, allnex© polymer-in-water, and oil-in-water emulsions, among others, while keeping its versatility in droplet size and frequency.
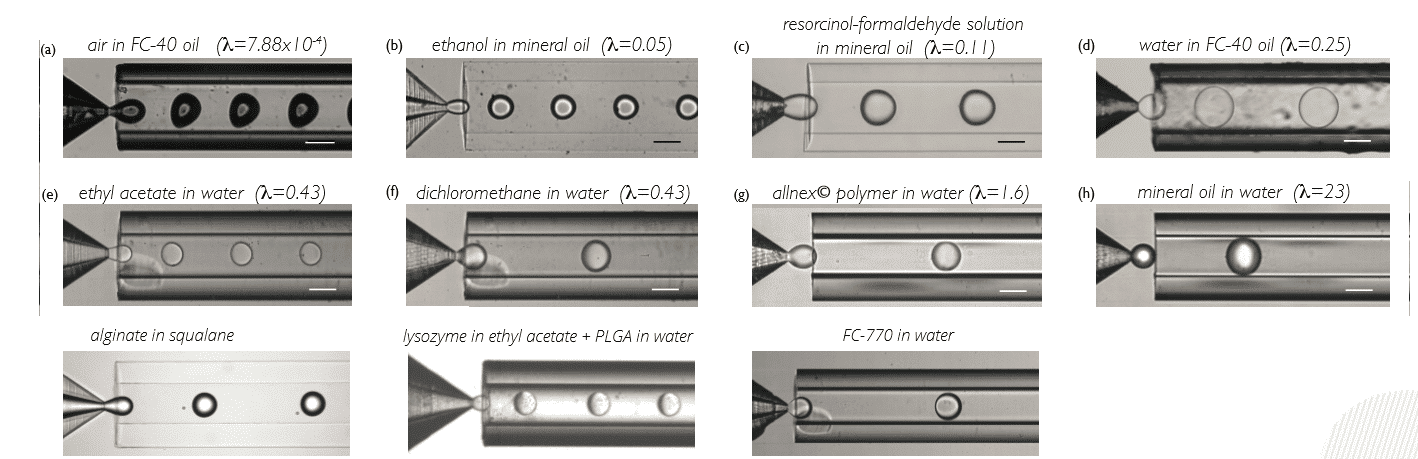
Figure 8: Experimental images of droplet generation in the dripping regime using the Raydrop with different couples and various pairs of fluids in a wide range of viscosity ratios. The scale bar is 100 μm. λ is the viscosity ratio.
Conclusion
The authors demonstrated several advantages of the Raydrop for capillary-based droplet production, including:
- high monodispersity ensured by the dripping regime,
- robustness of the fabrication technique,
- optimization capabilities based on numerical modeling,
- and the universality of the configuration.
To learn more about Secoya and the Raydrop, read our interview with Benoît Scheid, co-author of the aforementioned article.
Expertises & Resources
-
Microfluidic Application Notes 1-10 microns PLGA microsphere production using the RayDrop Read more
-
Microfluidics Article Reviews Solid lipid nanoparticles for biologics and drug encapsulation Read more
-
Microfluidic Application Notes PLGA nanoparticle synthesis using 3D microfluidic hydrodynamic focusing Read more
-
Microfluidic Application Notes Alginate Microbeads Production Read more
-
Microfluidic Application Notes Liposome Nanoparticle Synthesis Read more
-
Microfluidic Application Notes PLGA Microparticles Synthesis Read more