Microfluidic pressure control for organ-on-a-chip applications: A comprehensive guide
Organ-on-Chip technology is an emerging domain that’s working to revolutionize research fields and the pharmaceutical industry. The technology is an alternative technique to animal experimentation and that is gaining attention in the scientific community. One major component of a successful Organ-on-Chip model is a well-controlled microfluidic flow, allowing for accurate reproduction of an organ microenvironment. Several technologies are currently used for flow control, but certain criteria should be considered when choosing the right one. Microfluidic pressure control presents several advantages for OOC applications, including high stability and high responsiveness of the flow rate.
This comprehensive guide will cover several essential definitions, advantages, and disadvantages of different technologies, considerations for choosing the right technology, and examples of OOC using Fluigent devices.
Overview of microfluidic pressure control for organ-on-a-chip applications
Definition of microfluidics
Microfluidics is the science that studies the behavior, manipulation, and control of small volumes of fluids (picolitres-microliters) at the micrometer scale. It involves channels and structures with dimensions ranging from micrometers to tens of micrometers (1). It offers advantages such as reduced sample and reagent consumption, rapid analysis and processing times, high sensitivity, and the ability to perform complex and multiplexed assays in a miniaturized format. It has emerged as a powerful technology for various applications, including organ-on-chip systems.
Definition of Organ-on-chip
Organ-on-chip (OOC) is a rapidly evolving field that aims to mimic the structure and function of human organs in a microscale device (2). OOC devices typically consist of microfluidic channels lined with cells that are organized to replicate the cell types found in a specific organ. By recreating the microenvironment and cellular interactions of an organ, OOC technology provides a more physiologically relevant model for studying organ function, disease mechanisms, and drug responses compared to traditional two-dimensional cell cultures or animal models.
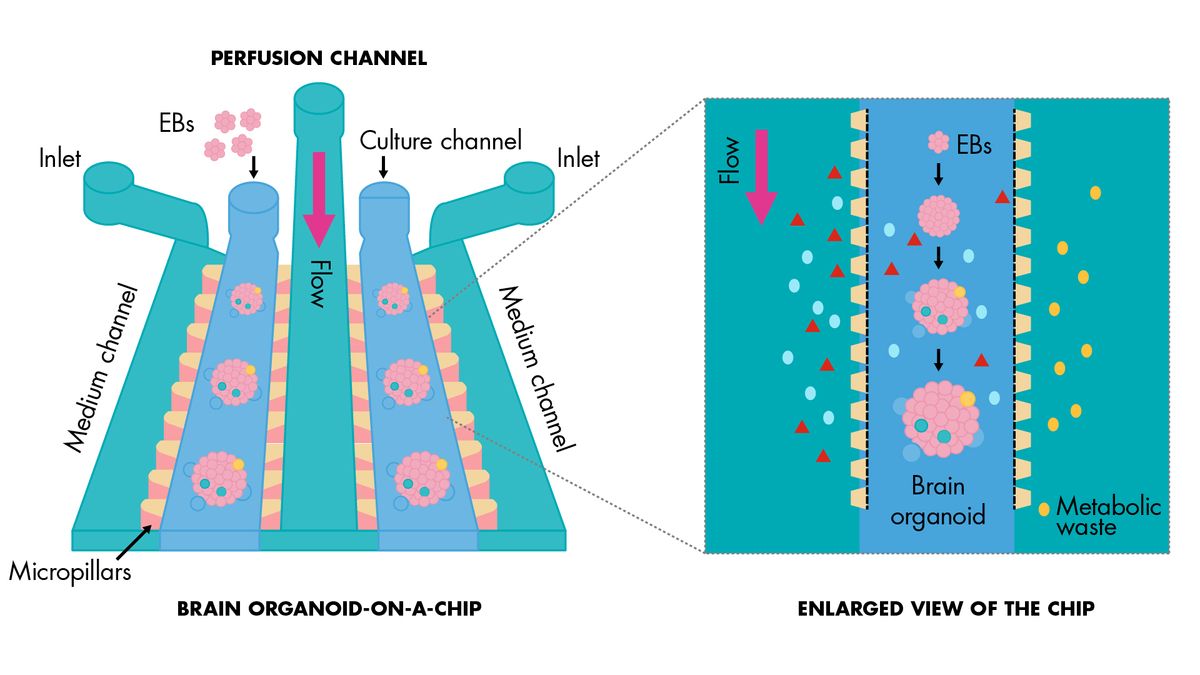
Figure 1: Example of an OOC: Brain organoid on chip combining biology, microfluidics, and recreation of microenvironment.
Common microfluidic technologies used for OOC applications
Mimicking the physiological microenvironment of living organs employing OOC devices is essentially achieved by implementing controlled liquid perfusion systems to simulate the flow of nutrients, drugs, and other substances through the microfluidic system. Several microfluidic flow control technologies are commonly used by researchers, each having their advantages and their disadvantages (Figure 2).
Peristaltic Pumps
Peristaltic pumps use positive displacement to squeeze a leak-proof flexible tube to create a pulsatile flow of liquid through the tube. It is an accessible and easy-to-set-up system used in biological fields, as it is compatible with various types of fluids and presents a low risk of contamination since the liquid only contacts the tubing. However, its flow rate control and pressure capabilities are relatively low, and its pulsatile aspect may not be suitable for certain applications.
Syringe Pumps
A syringe pump is a motor-driven precision pump that uses one or more syringes to deliver precise and accurate amounts of fluid. They are generally user-friendly, with intuitive control interfaces for programming and adjusting parameters such as flow rate, volume, and infusion rates. They can provide both continuous and intermittent flow, depending on the programming settings. This feature is useful for experiments or procedures that require specific flow patterns. Despite their advantages, syringe pumps have a few limitations such as low flow rate control and responsiveness, limited volume of the syringe which will require periodic refilling steps, and an increased risk of contamination as the fluid is in direct contact with the syringe.
Pressure generators
Pressure generators utilize external pneumatic or hydraulic systems to generate pressure and drive the liquid flow through the microfluidic system. Among multiple advantages, high flow rate stability and fast responsiveness are the most pertinent features needed for microfluidic pressure control for OOC applications. They can also provide continuous and pulsatile flow options, depending on the application, and can be easily integrated into multi-organ systems. This technology is more complex than the latter ones, as it requires additional equipment and a sophisticated setup.
It’s important to note that the choice of liquid perfusion technology depends on the specific requirements of the organ-on-chip application, such as the desired flow rate, pressure, pulsatility, and compatibility with the cells or tissues being studied. Researchers often select the most appropriate technology based on the advantages and disadvantages mentioned above and tailor it to their experimental needs.
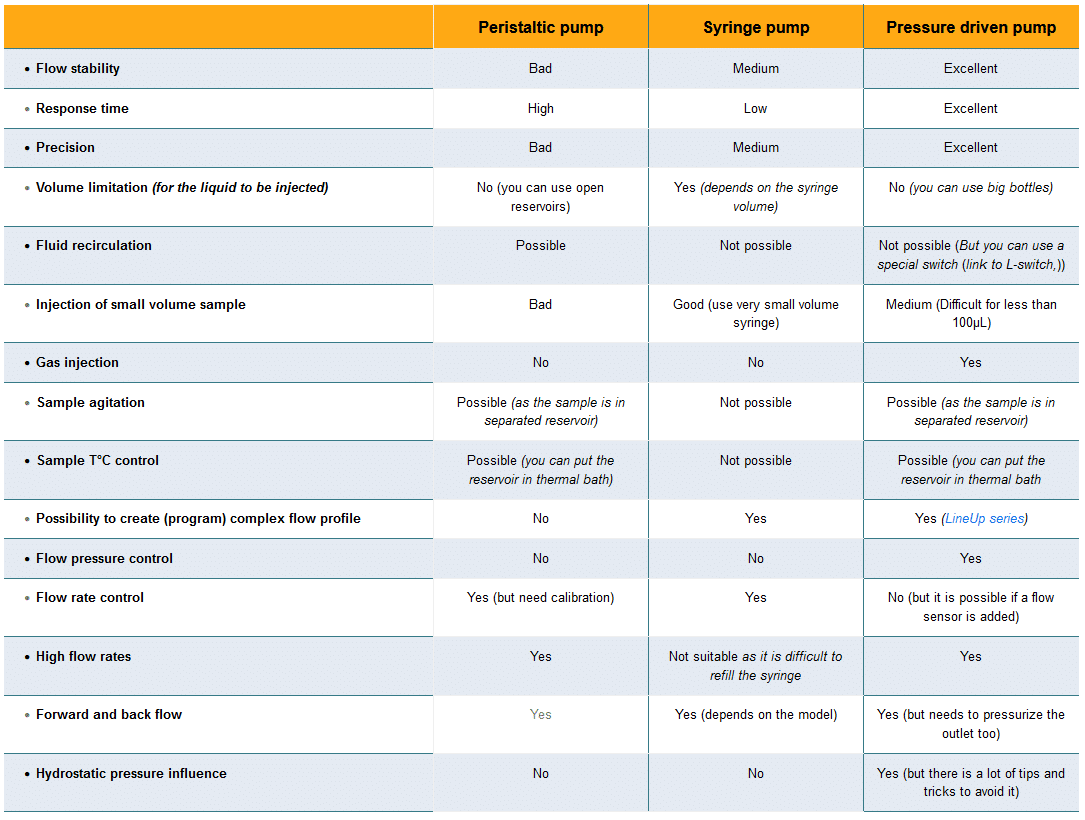
Figure 2: Advantages and disadvantages of different microfluidic technologies used for OOC.
Why use microfluidic pressure control for Organ-on-Chip applications?
Microfluidic pressure control plays a crucial role in the success of organ-on-chip applications. It enables researchers to precisely control and manipulate the flow rates, shear stresses, and mechanical forces experienced by cells and tissues within the microfluidic device. By mimicking the in-vivo physiological conditions, microfluidic pressure control enhances the accuracy and relevance of organ-on-a-chip experiments. Pressure control is closely related to flow control, so by modulating the pressure, flow rates can be accurately adjusted, enabling precise control over fluid dynamics and directing the flow to specific regions of the chip. Accurate flow control allows for recreating complex fluidic environments and mimicking microenvironmental cues (such as shear stress and pressure gradients), affecting the behavior and function of cells. Moreover, precise pressure control is essential for controlled drug delivery and perfusion within the organ-on-a-chip device. It allows for controlled exposure of cells and tissues to drugs, toxins, or other substances, enabling the assessment of their effects on organ function and response.
Understanding microfluidic pressure control
Components and Mechanisms
Within microfluidics systems, various components play crucial roles in ensuring precise fluid control and pressure management.
- Pressure sources: employed to generate controlled fluid flow and pressure within microfluidic systems. Common types of pumps used in microfluidics include syringe pumps, peristaltic pumps, and pressure-driven pumps. They enable the precise delivery of fluids and the generation of desired flow rates and pressures.
- Pressure regulators: they ensure that the pressure remains constant or follows a predefined profile by using feedback from pressure sensors to adjust the flow rate and maintain the desired pressure level.
- Pressure Sensors: used to measure the pressure within microfluidic channels. These sensors can be integrated into the chip or connected externally to monitor and provide feedback on the pressure conditions in real-time. They convert the pressure exerted by the fluid into electrical signals, which are then used by the pressure regulators to make necessary adjustments. Common types of pressure sensors used in microfluidics include piezoresistive and capacitive sensors.
- Valves: crucial components of a microfluidic system that regulate fluid flow within microfluidic channels. They can be mechanical, pneumatic, or electrostatic, and they control the opening and closing of specific channels to direct the flow of fluids as required. Valves allow researchers to create complex fluidic networks and dynamic flow patterns within OOC devices.
- Feedback Control Systems: use information from pressure sensors or other sensors in the systems to adjust the pressure or flow rates in real-time. These systems provide a closed-loop control mechanism, enabling the device to maintain desired pressure conditions.
By integrating these components, researchers can effectively use microfluidic pressure control (Figure 3) via Fluigent systems to create accurate fluidic environments necessary for organ-on-chip applications.
Considerations for Organ-on-Chip Applications
Media perfusion is a hallmark of OOC devices, serving as a circulatory system mimic that maintains a concentration gradient for nutrient and waste convective transport (3). The choice of the microfluidic liquid perfusion system to connect to the organ-on-a-chip needs to be considered based on several factors:
- Flow rate, pressure control and pulsatility
Reproducing a physiological microenvironment requires mimicking the same conditions in-vivo, depending on the studied organ. For example, lung-on-chip models, that aim to mimic the breathing motion and airflow in the lungs, may require low-to-moderate flow rates (a few microliters per minute) and gentle pressure conditions to simulate breathing dynamics and the alveolar microenvironment accurately. Other applications such as heart-on-chip models often require pulsatile flow to mimic the rhythmic contraction of the heart. They may need higher flow rates (tens to hundreds of microliters per minute) and moderate-to-higher pressure capabilities to generate pulsatile flow patterns that replicate the physiological pumping action of the heart.
- Cell viability and shear stress
OOC systems include the culture of living cells and tissues that are sensitive to mechanical forces and fluidic conditions. Excessive shear stress can impact cell viability, proliferation and functionality, thus, it is important to consider the shear stress levels generated by the pump type and select one that minimizes potential damage to the cells while maintaining adequate fluid flow. For example, when working with neuronal cells in brain models that are sensitive to shear stress, it is crucial to carefully regulate flow rates and avoid high shear stress conditions that could disrupt cell networks or induce cell damage.
- Contamination risk
Maintaining a sterile environment is crucial in OOC applications. Some pump designs allow for contact between the fluid and the pump components, as this can increase the risk of contamination. Pumps that minimize or eliminate contact between the fluid and pump components are preferred for OOC applications.
- Integration and compatibility
Today, OOC models are aiming to replicate interactions between different organs within the human body. For example, a liver-on-chip and lung-on-chip may be connected to study drug metabolism and toxicity effects. The pumps and microfluidic setups of each organ model need to be designed to enable fluid flow and communication between the different chips. Moreover, some OOC models may also include pH levels, oxygen levels, and electrical activity sensors, hence the importance of considering compatibility with the microfluidic pressure controller. Finally, long-term cultures and imaging/analysis techniques are crucial for any OOC experiment, therefore it is important to consider the compatibility between the microfluidic platform and the Bio-cabinet/imaging/analysis equipment, including positioning, sample accessibility, and optical clarity.
Applications in Organ-on-Chip
In recent years, researchers have made significant progress in developing organ-on-a-chip models for various organs. Examples include kidney on a chip (4), lung on a chip (5), heart on a chip (6), skin on a chip (7), pancreas on a chip (8), brain on a chip, gut on chip, and blood-brain barrier on a chip (9). The use of microfluidic technologies has been crucial in overcoming several limitations associated with these models, such as:
- Replicating the organ’s exposure to fluids in a controlled and directional manner
- Facilitating the flow of nutrients into the chip and the removal of waste products
- Establishing gradient flows and allowing for more precise dosing studies
- Enabling the layering of multiple cell types to mimic the complex structure of organs
- Using human-derived cells in organ-on-a-chip studies thus providing a more physiologically relevant model compared to animal models
In summary, organ-on-a-chip microfluidic devices have revolutionized research in several areas, including high throughput drug screening, single-cell analysis, cell-cell interactions, and the study of various biological processes and diseases. These advancements have opened new possibilities for understanding human physiology and developing more effective therapies.
Successful Organ-on-chip examples using Fluigent’s systems
Fluigent’s microfluidic pressure control systems have been used by many researchers in their investigations on novel Organ-on-a-chip models.
- Kidney-on-chip
MJ Hoogduijn’s team (10) performed the first vascularization of kidney organoids in HUVEC co-culture conditions using a microfuidic organ-on chip system. They first cultured kidney organoids (Figure 4) that presented nephron structures on chip and demonstrated an increased maturation of endothelial populations based on a colocalization analysis of endothelial markers (MCAM & PECAM) compared to transwell organoid cultures (Figure 5). They also performed co-cultures of these organoids along with human umbilical vein endothelial cells (HUVECs). These cells were able to migrate and proliferate inside the organoid tissue, and to interconnect with endogenous endothelial cells, which led to the formation of structures presenting an open lumen resembling vessels (Figure 6). They used Fluigent’s microfluidic pressure controller, FlowEZ, at a constant rate of 3 µL/min for 48 h after static culture.
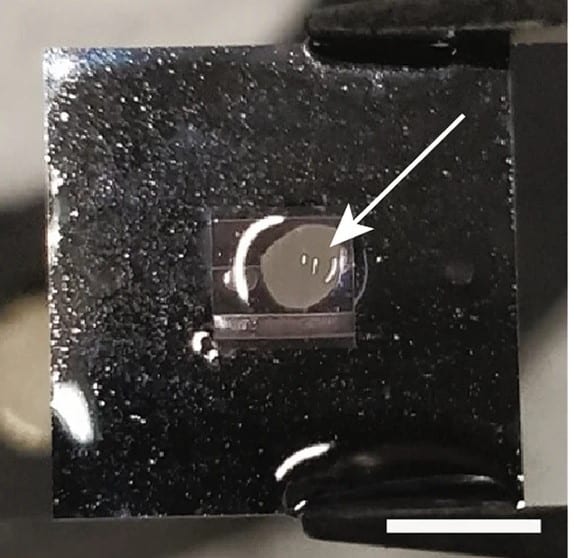
Figure 4: Kidney organoid-on-a-chip
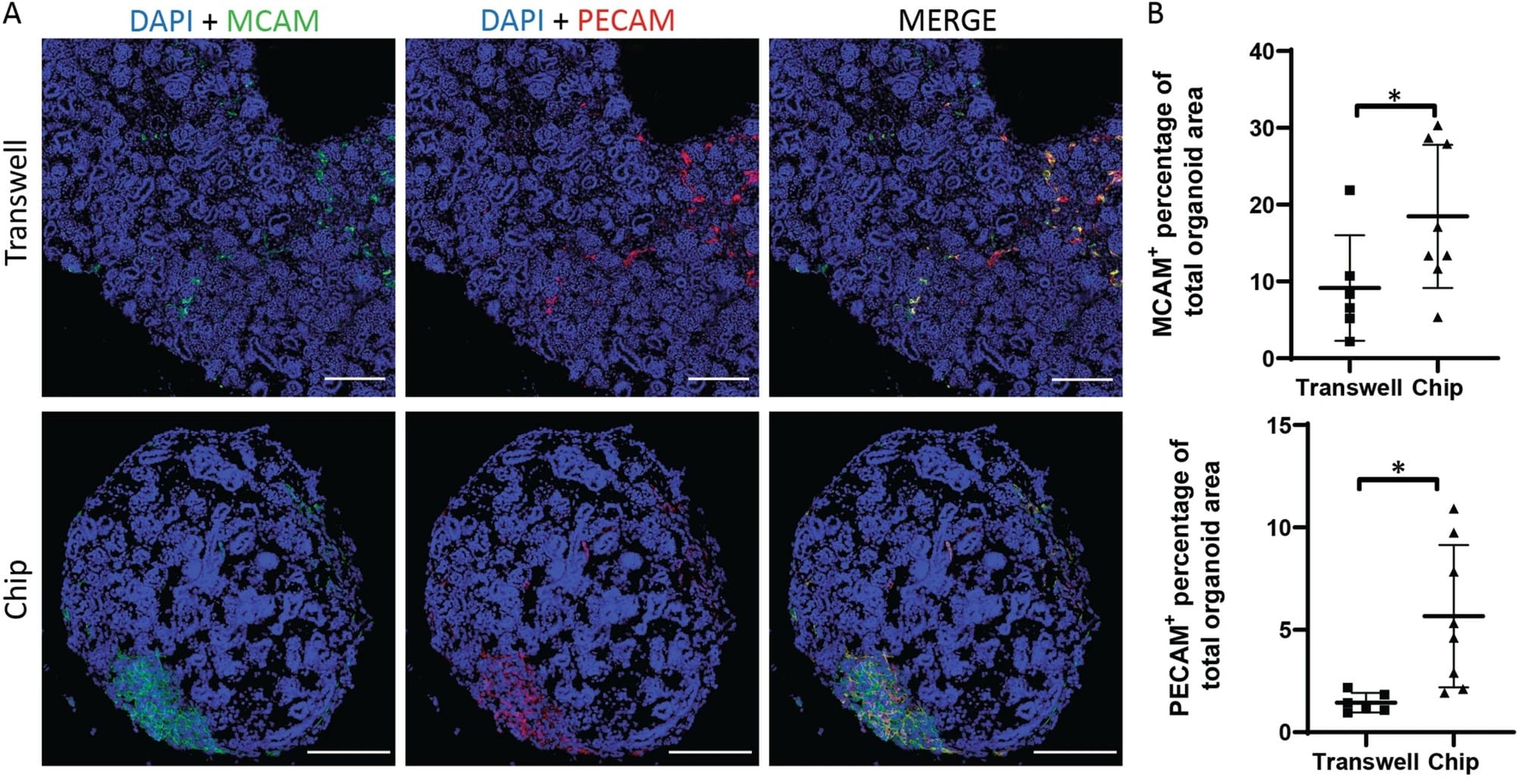
Figure 5: Kidney organoids cultured on a chip show expanded EC populations and directed endothelial maturation patterns
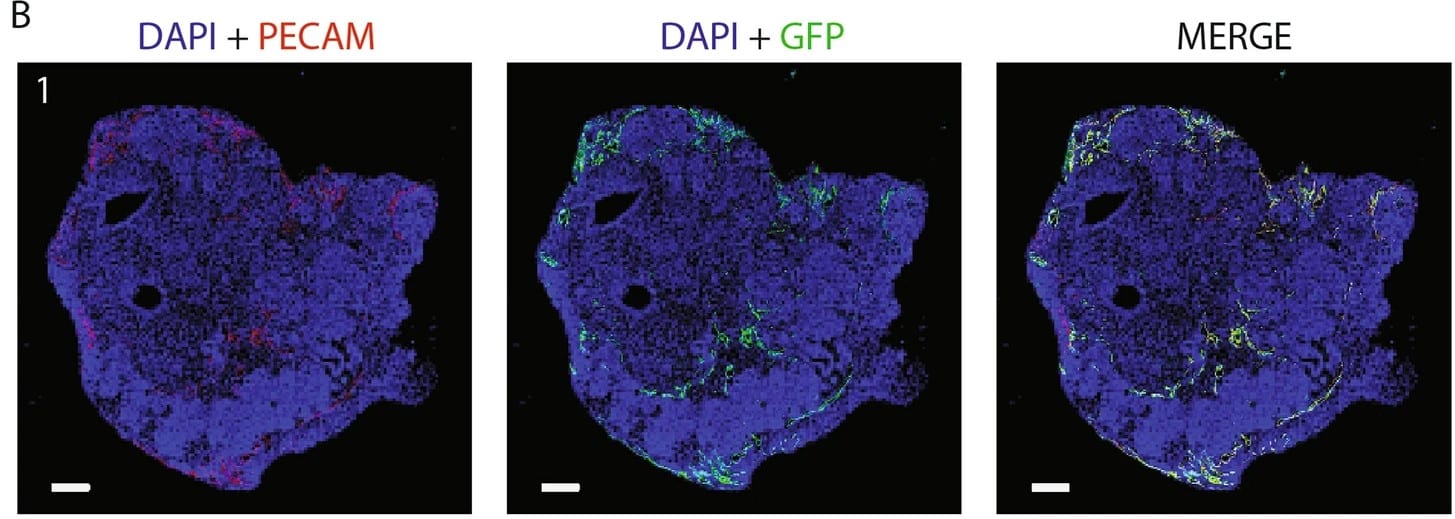
Figure 6: Vascular structures derived from the GFP+ HUVECs integrated in the organoid tissue.
- Blood vessel-on-a-chip
Valeria Orlova’s team (11) developed a fluidic circuit board (FCB) for simultaneous perfusion of up to twelve 3D-VoCs using a single set of control parameters (Fluidic circuit composed of two FlowEZ, 1 Linkup and a flow sensor, by Fluigent) (Figure 7). The throughput of conventional 3D systems is one channel at a time due to difficulty in controlling dynamic microenvironmental cues such as wall shear stress (WSS) and circumferential strain (CS) in highly variable individual 3D-VOCs (intrinsic diameter).
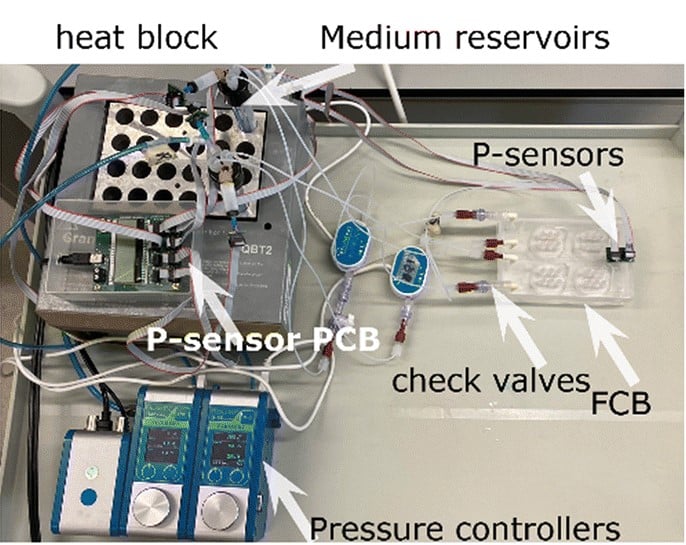
Figure 7: Photograph of the tested fluidic circuit board connected to the external medium reservoirs in a heat block.
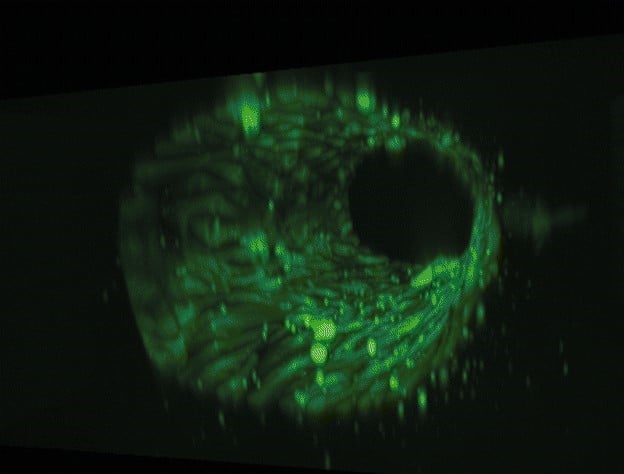
Figure 8: 3D reconstruction of a blood vessel on a chip.
- Cancer-on-chip
Van Gent’s team developed a Cancer-on-a-Chip microfluidic platform (Figure 9) for the assessment of patient treatment responses using tumor tissue slices under controlled growth conditions (12). Their microfluidic pressure control system consists of Fluigent’s MFCS-EZ (Figure 10). They were able to maintain cell viability and sustain proliferation of breast and prostate cancer PDX tumor slices for up to 14 days. They also studied the tumor slices response to cisplatin and antidrogen chemotherapeutic treatment. Their platform could be used for future studies including biopsies of patient tumors that will be treated with the same chemotherapy.
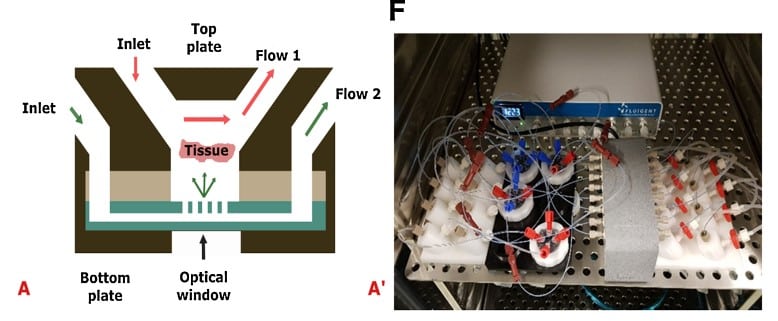
Figure 9: (A) Cross-section of the Cancer-on-a-Chip system illustrating the diffusion and perfusion toward the tissue slice.
Figure 10: (A’) The Cancer-on-a-Chip platform connected to a Fluigent Microfluidic flow control system further connected to flowrate sensors (Fluigent FLOW UNIT-S) using Fluigent’s software throughout the culture period.
- Cartilage-on-a-chip
Séverine le Gac’s team developed a cartilage on chip model using our microfluidic control system (MFCS-EZ, 2 switches and a switchboard) to simulate how chondrocytes react to external stimuli (mechanical or chemical) and to understand processes triggering cartilage diseases like osteoarthritis (13) (Figure 11). This setup notably implements mechanical stimulation on 3D cell cultures and studies the cell response to these stimulations in situ, while also creating dynamic culture conditions.
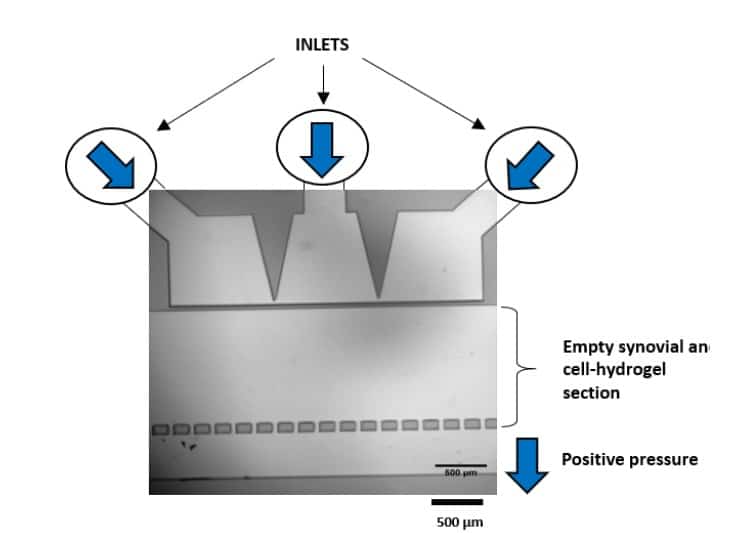
Figure 11: Picture of the culture chamber and the three actuation chambers to induce external mechanical stimulus on the chondrocytes.
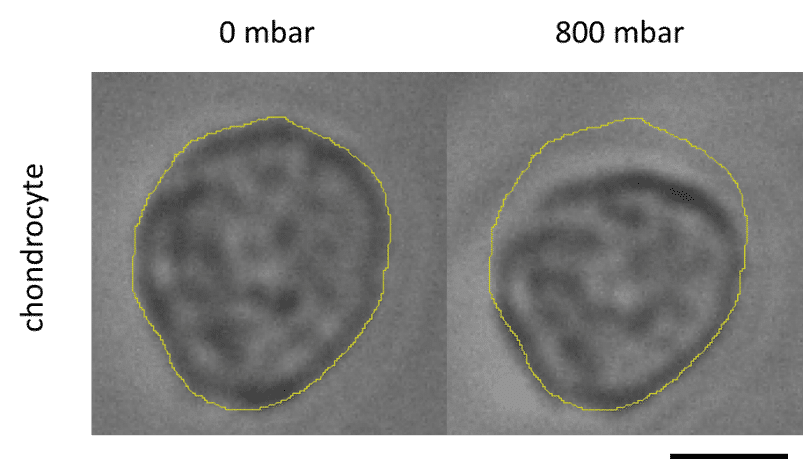
Figure 12: Chondrocyte deformation after mechanical stimulation.
OOAC Webinar Recording: Explore Topics of Interest
Webinar – Organ on chip Towards the next generation of cell culture platforms
Overview
Fluigent and Beonchip are partnering to offer a complete solution for organ on-chip. This webinar will first introduce Beonchip, their chips and the numerous applications that can be performed.
The second part will focus on flow control systems. Although often considered secondary, flow control is as important as chip design as cells are highly sensitive to mechanical forces. Results demonstrate how cells are affected by peristaltic pumps compared to pressure control systems.
Organ on chip study is an emerging field that brings substantial benefits compared to conventional cell culture. In many labs, considerable effort is put in choosing the right chip design, but the impact of flow control is still undetermined.
It is our intent to create awareness of the importance of flow and its effects on one’s studies.
Read our expertise page to know more about the benefits of flow control in cell culture, learn more about our products and those of our partners (Beonchip) as well as reach out to our team of experts to find a solution that best meets your needs.
SPEAKERS :
Marine Verhulsel, Product manager, Fluigent
Luis Serrano, Head of sales and business development, BEONCHIP S. L.
Introducing Omi: Revolutionizing fluid control for Organ-on-a-Chip applications
Fluigent is contributing to the revolutionary field of Organ-on-a-Chip studies by introducing Omi (Figure 13), our cutting-edge organ-on-chip platform. Omi was meticulously designed to transform the way fluids are handled in research and industrial laboratories and facilities. The system offers unparalleled precision, versatility, and ease of use. With its ultra-precise microfluidic pressure control, intuitive user interface and advanced software, Omi allows users to create complex and long-term fluidic protocols. The system’s modular design enables seamless integration into existing experimental setup, providing a flexible and scalable solution tailored to specific scientific needs. Omi offers precise pressure control, flow rate regulation, and automated fluid switching, ensuring optimal performance and reproducibility in experiments. It can perform different protocols based on experimental needs, including perfusion, recirculation, injection, and/or sampling. It’s suitable for testing multiple types of drugs, toxins, and metabolites.
Features include:
- Compatability: can be used with any kind of microchip via the included adaptor
- A compact and portable design for easy transitions between the incubator, hood, and microscope while maintaining a running flow.
- Ability to be controlled remotely via WIFI connectivity and the iOS/Android Omi application
- Data storage in the cloud for easier accessibility
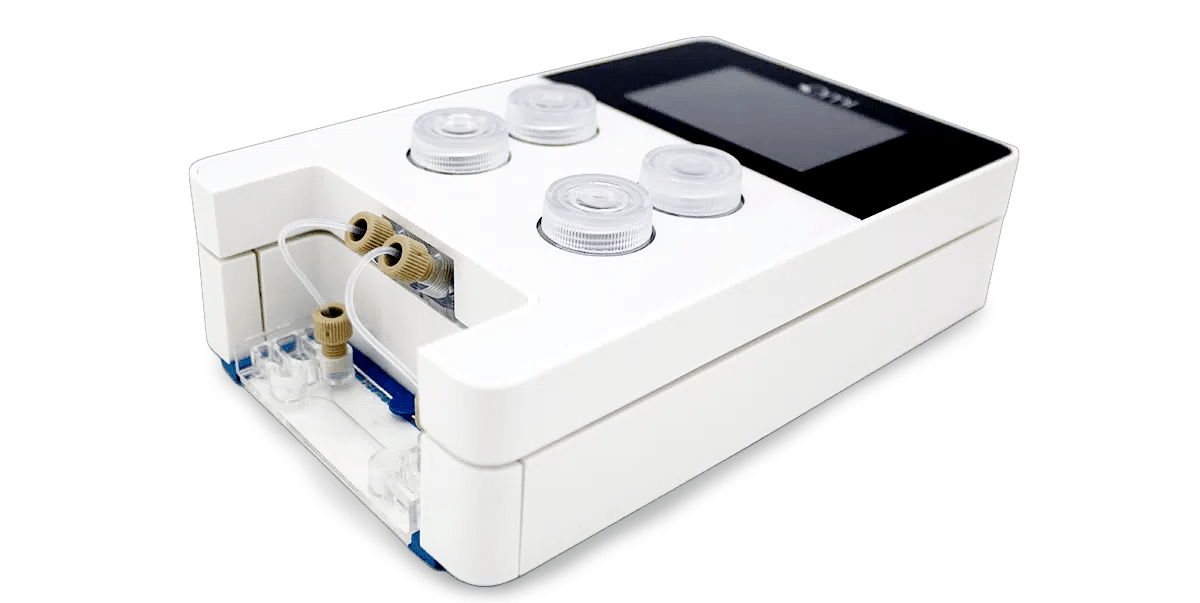
Figure 13: Fluigent’s Organ-on-a-Chip recirculation platform, Omi.
Related Products
Expertises & Resources
-
Microfluidics case studies A microfluidic Artery-on-a-Chip using Fluigent’s Microfluidic Flow Control System, the MFCS Read more
-
Microfluidics case studies Creating a Microfluidic Cancer-on-Chip Platform using Fluigent’s High Throughput Cell Perfusion Pack Read more
-
Microfluidic Application Notes Long-term fluid recirculation system for Organ-on-a-Chip applications Read more
-
Microfluidics case studies CNRS/UTC: study of a liver-on-a-chip model Read more
-
Microfluidics Article Reviews Human Blood Brain Barrier (BBB) permeability -on-chip assessment Read more
-
Microfluidics White Papers Microfluidic white paper – A review of Organ on Chip Technology Read more
-
Expert Reviews: Basics of Microfluidics Why is a controlled shear-stress a key parameter of your microfluidic experiments? Read more
-
Microfluidic Application Notes Development of a human gut-on-chip to assess the effect of shear stress on intestinal functions Read more
-
Expert Reviews: Basics of Microfluidics How to choose a microfluidic chip Read more
-
Microfluidics White Papers An exploration of Microfluidic technology and fluid handling Read more
-
Microfluidic Application Notes Peristaltic Pump vs Pressure-Based Microfluidic Flow Control for Organ on Chip applications Read more
-
Microfluidic Application Notes Cartilage-on-a-chip, an example of complex mechanical stimulation using Fluigent’s technology Read more
-
Expert Reviews: Basics of Microfluidics Mimicking in-vivo environments: biochemical and biomechanical stimulation Read more
-
Expert Reviews: Basics of Microfluidics Microfluidic Flow Control: Comparison between peristaltic, syringe and pressure pumps for microfluidic applications Read more
-
Expert Reviews: Basics of Microfluidics Microfluidics overview: History and Definition Read more
References
1. Whitesides, G.M. The origins and the future of microfluidics. Nature 2006, 442, 368–373.
2. Leung, C.M., de Haan, P., Ronaldson-Bouchard, K. et al. A guide to the organ-on-a-chip. Nat Rev Methods Primers 2, 33 (2022). https://doi.org/10.1038/s43586-022-00118-6.
3. Leung, C.M., de Haan, P., Ronaldson Bouchard, K. et al. A guide to the organ-on-a-chip. Nat Rev Methods Primers 2, 33 (2022). https://doi.org/10.1038/s43586-022-00118-6.
4. S.K. Jeonghwan Lee, Kidney-on-a-Chip: a new technology for predicting drug efficacy, interactions, and drug-induced nephrotoxicity, Curr. Drug Metab. 19 (2018) 577–583.
5. P. Zamprogno, S. Wüthrich, S. Achenbach, Second-generation lung-on-a-chip with an array of stretchable alveoli made with a biological membrane, Commun. Biol. 4 (2021) 168.
6. H. Liu, O.A. Bolonduro, J.J. Ning Hu, A.A. Rao, B.M. Duffy, Z. Huang, L.D. Black, B.P. Timko, Heart-on-a-Chip model with integrated extra- and intracellular bioelectronics for monitoring cardiac electrophysiology under acute hypoxia, Nano Lett. 20 (2020) 2585–2593.
7. B. Luk´ acs, A. ´ Bajza, D. Kocsis, A. Csorba, I. Antal, K. Ivan, ´ A.J. Laki, F. Erdo, Skinon-a-Chip device for ex vivo monitoring of transdermal delivery of drugs—design, fabrication, and testing, Pharmaceutics 19 (2019) 445.
8. K. Shik Mun, K. Arora, Y. Huang, Patient-derived pancreas-on-a-chip to model cystic fibrosis-related disorders, Nat. Commun. 10 (2019) 3124.
9. L.I. Ilaria Raimondi, Marta Tunesi, Manola Comar, Diego Albani, Carmen Giordano, Organ-On-A-Chip in vitro models of the brain and the bloodbrain barrier and their value to study the microbiota-gut-brain axis in neurodegeneration, Front. Bioeng. Biotechnol. (2020).
10. Creating a kidney organoid‑vasculature interaction model using a novel organ‑on‑chip system Amanda Bas‑Cristóbal Menéndez 1,2*, Z. Du1 , T. P. P. van den Bosch3 , A. Othman4 , N.Gaio4 , C. Silvestri4 , W. Quirós4 , H. Lin5 , S. Korevaar1 , A. Merino1 , J. Mulder2,6 & M. J. Hoogduijn1.
11. Multiplexed fluidic circuit board for controlled perfusion of 3D blood vessels-on-a-chip† Mees N. S. de Graaf, a Aisen Vivas, bc Dhanesh G. Kasi,ade Francijna E. van den Hil,a Albert van den Berg, c Andries D. van der Meer, b Christine L. Mummery ab and Valeria V. Orlova *a.
12. (A Microfluidic Cancer-on-Chip Platform Predicts Drug Response Using Organotypic Tumor Slice Culture Sanjiban Chakrabarty1,2, William F. Quiros-Solano3,4, Maayke M.P. Kuijten1,5, Ben Haspels1 , Sandeep Mallya6 , Calvin Shun Yu Lo1 , Amr Othman4 , Cinzia Silvestri4 , Anja van de Stolpe7 , Nikolas Gaio4 , Hanny Odijk1 , Marieke van de Ven8 , Corrina M.A. de Ridder9 , Wytske M. van Weerden9 , Jos Jonkers8 , Ronald Dekker3,7, Nitika Taneja1 , Roland Kanaar1,5, and Dik C. van Gent1.
13. Emulating the chondrocyte microenvironment using multi-directional mechanical stimulation in a cartilage-on-chip† Carlo Alberto Paggi,ab Jan Hendriks,a Marcel Karperien *a and Séverine Le Gac *b