Controlled Flow System for Altering Cardiac Mechanical Load in Zebrafish Model
This case study introduces a novel microfluidic approach using zebrafish larvae model for real-time, in vivo analysis. By injecting a controlled volume upstream of the heart, J. Baillie et al. induced acute atrial dilation while measuring heart rate and stroke area.
The obtained results revealed that early-stage adaptation relies on mechano-mechanical coupling, unlike in adults. Enabled by Fluigent’s pressure-based flow control, this method offers a powerful tool for cardiac development and mechanobiology research.
A Paper from Dalhousie University
Paper: Baillie, J. S.; Gendernalik, A.; Garrity, D. M.; Bark, D.; Quinn, T. A. The in Vivo Study of Cardiac Mechano-Electric and Mechano-Mechanical Coupling during Heart Development in Zebrafish. Front. Physiol. 2023, 14, 1086050.
This study is the result of a collaboration between the Colorado State University, the Washington University in St. Louis, and the Cardiac, Autoregulation & Arrhythmias Laboratory at Dalhousie University. Led by Dr. Alexander Quinn, the lab is dedicated to understanding the intrinsic regulation of cardiac function and its role in deadly arrhythmias to advance the prevention and treatment of cardiovascular disease. Their research focuses on uncovering key autoregulation mechanisms, understanding their impact on heart rhythm, and applying these insights to develop targeted anti-arrhythmic therapies.

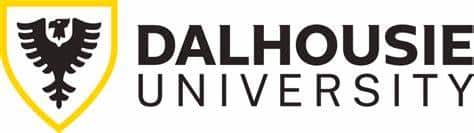
Testimonial
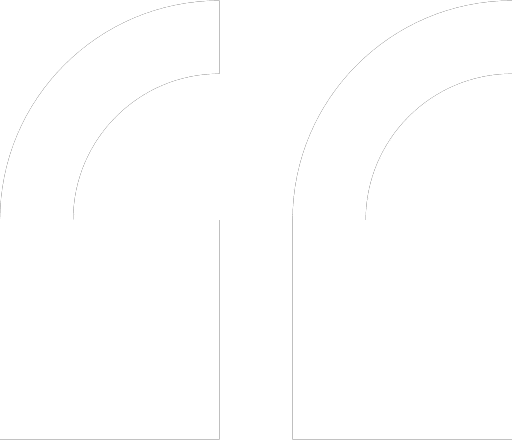
“The FlowEZ pressure controller, Flow Unit flow rate sensor, and OxyGEN software from Fluigent made setting up a custom microfluidics system for our specific needs quick and simple. It’s ease of use and user friendly implementation have allowed for precise and reliable control of cardiac mechanical load in both larval and adult zebrafish, enabling highly reproducible in vivo and in vitro investigations of mechanical responses critical for control of cardiac activity. We are excited about the physiological insights this system will provide as we continue to utilise this powerful technology. ”
Alex Quinn, Ph.D., Professor, Physiology & Biophysics and Biomedical Engineering, Dalhousie University
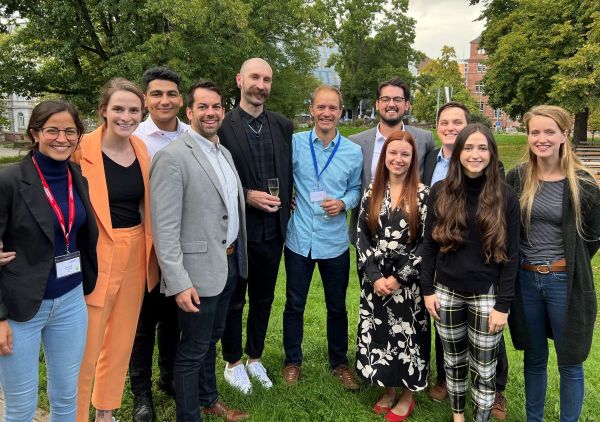
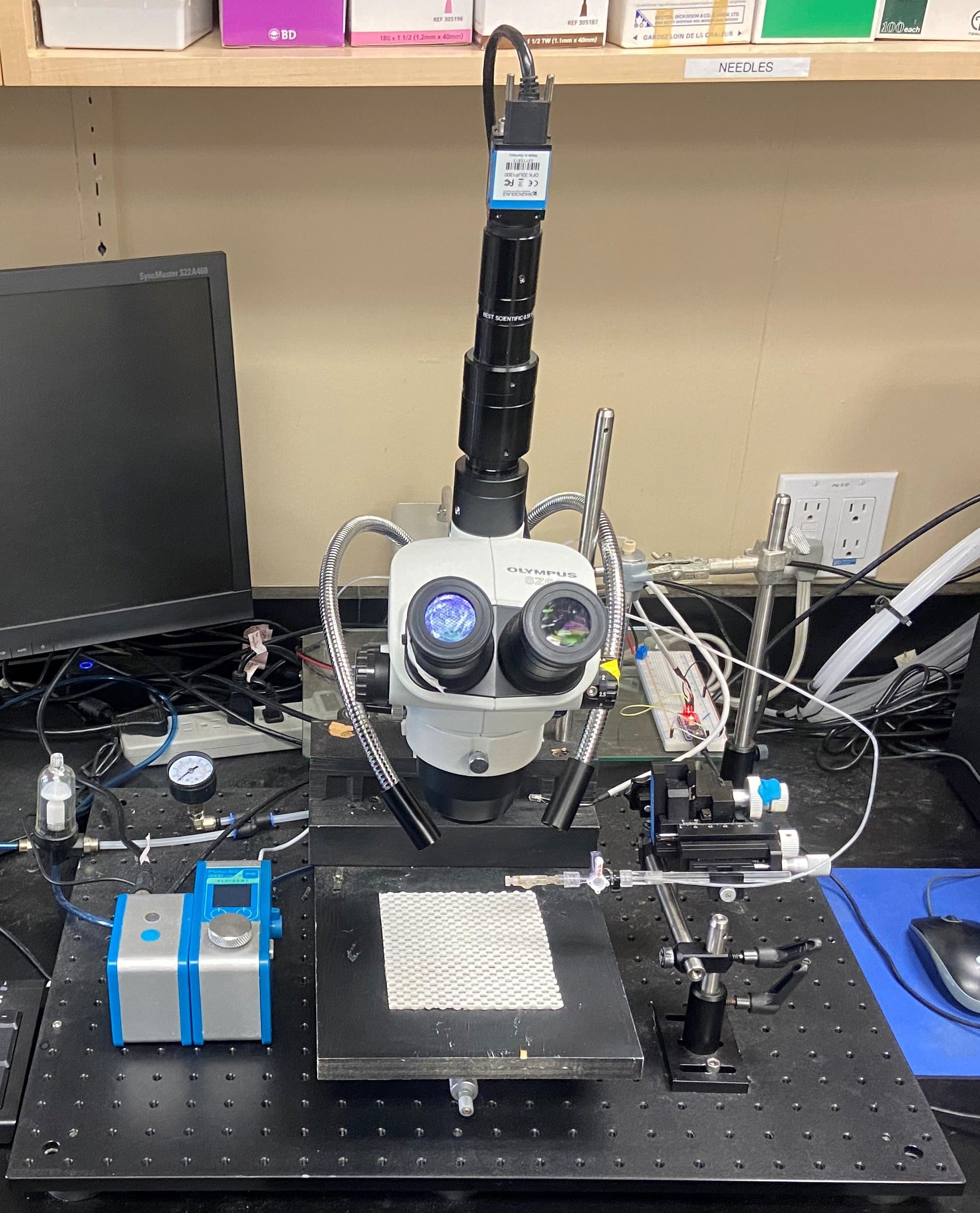
Why is Mechanical Load Critical for Cardiac Development and Function
The heart functions as an electrically driven pump, adapting to constant changes in mechanical load to regulate blood flow. In each heartbeat, cardiomyocytes contract and relax in response to preload (blood filling the heart) and afterload (resistance to ejection), adjusting cardiac output through mechano-electric (MEC) and mechano-mechanical (MMC) coupling. While these adaptive mechanisms are well understood in adults, their role in heart development remains unclear. 2,3
During embryogenesis, the heart evolves from a simple tube into a multi-chambered organ, driven by mechanical forces that shape cardiac structure, function, and electrical activity. Mechanical load influences electrophysiology, cell differentiation, and tissue growth, highlighting its importance in early cardiac development. However, the extent to which acute mechanical load changes drive functional adaptation in the developing heart remains an open question, making this a crucial area for further research. 4–6
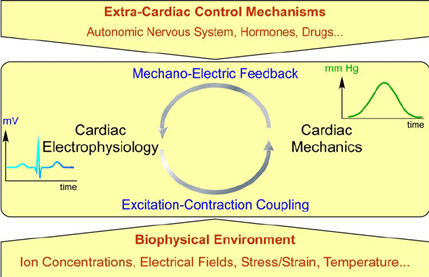
Figure 1: Overview of cardiac electromechanical integration. 6
Zebrafish as a Model for Mechanobiology and Cardiac Research Advancements
Zebrafish offer a powerful alternative experimental model for studying cardiac development and function, overcoming technical and physiological limitations seen in mammalian models. Unlike mammals, the transparent zebrafish embryo allows real-time visualization of developing organs, including the heart, in vivo. The zebrafish heart begins to beat and form major vessels within 48 hours of post-fertilization, making it ideal for studying early heart morphogenesis and mechanical load effects. Furthermore, the larvae embryo can survive significant cardiac dysfunction due to their unique oxygen diffusion system, enabling the study of severe phenotypes that would be destructive in other species. With a fully sequenced genome and easily manipulated genetics, zebrafish are a cost-effective model with relevant human cardiac gene orthologs and comparable heart function, including similar electrophysiology and responses to stretch. These advantages, along with optogenetic tools for precise control of cardiac activity, position zebrafish as an excellent model for investigating the role of mechanical forces in heart development.1,7–9
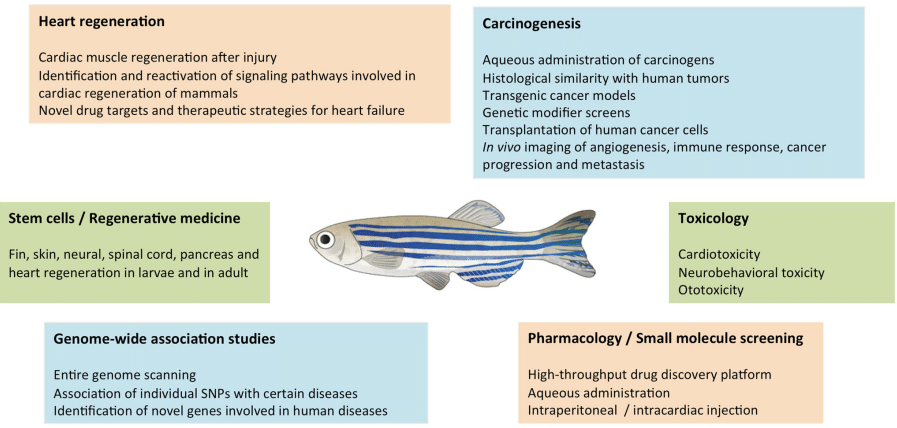
Figure 2: Zebrafish as a model organism for biomedical research.10
Aim of the Study
This study aims to investigate the acute effects of mechanical load on cardiac function during early development in zebrafish. By developing a novel experimental approach, the impact of preload alterations on the electrical and mechanical activity of the larval zebrafish heart are evaluated. The goal is to explore how mechanosensitive processes, such as MEC- and MMC-mediated responses, influence cardiac development, with a proof-of-concept study evaluating functional changes in the atrium at 48 hours post-fertilization.
Methodology: How to Set Up Precise Flow Control and Micro-cannula Injection for Zebrafish Model Studies
All procedures followed Dalhousie University’s animal care guidelines. 48 hpf zebrafish with a heart-specific eGFP marker were used for the study. Larvae embryos were anesthetized, immobilized in low-melting agarose, and positioned under a fluorescence microscope.
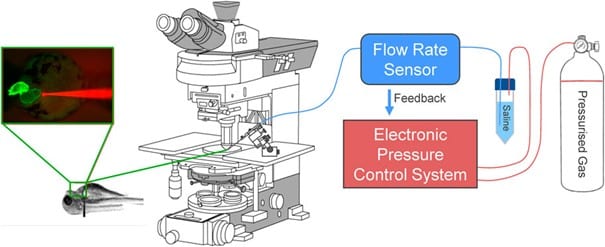
Figure 3: Electronic flow control system for acutely increasing cardiac preload in intact larval zebrafish.1
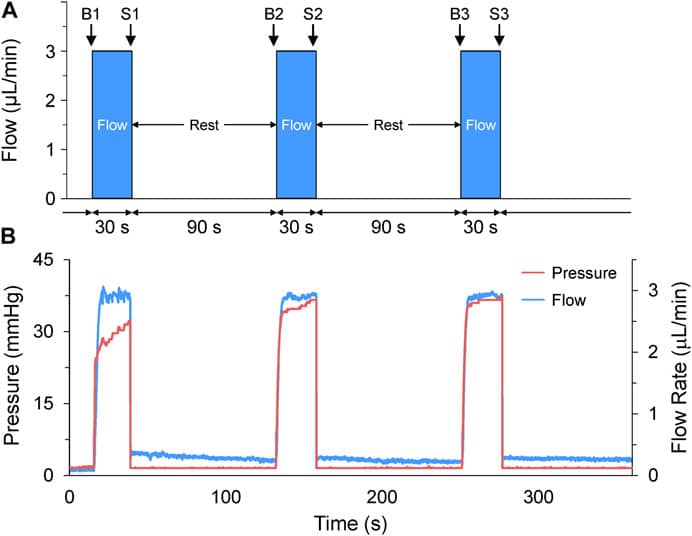
Figure 4: Acute volume loading protocol: (A) Three 30s saline injections (3 μL/min) with 90s rest periods; taken before (B1-B3) and after loading (S1-S3). (B) Pressure and flow monitored using pressure-flow control software.1
For precise control of preload, an advanced electronic flow control system was developed, building on previous hydrostatic pressurization techniques.11 This system uses Fluigent’s microfluidic pressure controller (FlowEZ, 2 bar) and flow rate sensor (Flow Unit M) to monitor and control flow rates enabling real-time adjustments of atrial preload.
Micro-cannulas (4 μm tip diameter) were inserted into the common cardinal vein of the larvae embryo to inject saline solution, inducing controlled atrial dilation. Injection protocols were optimized using Fluigent’s OxyGEN software to achieve a ~25% increase in atrial end-diastolic area. The optimal combination was found to be a cannula flow of 3 μL/min for 30 s. The heart’s functional responses, including heart rate and atrial dimensions, were monitored through high-speed video and analyzed using custom Matlab routines to calculate parameters such as heart rate (HR), atrial stroke area (SA), and cardiac output (COA).
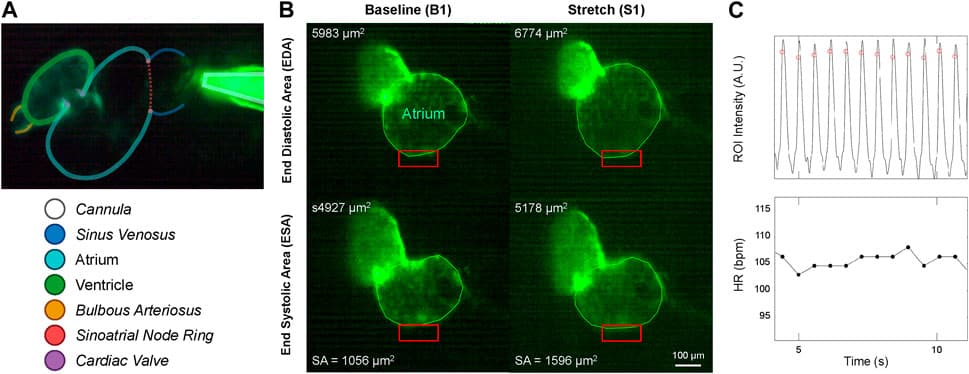
Figure 5: Micro-cannula placement and atrial dilation: (A) In vivo image of zebrafish heart with micro-cannula. (B) Heart videos analyzed in Matlab to calculate EDA, ESA, and stroke area (SA).1
Proof-of-Concept: Zebrafish Atrial Response to Increased Preload in Early Development
Acute volume loading resulted in a transient, three-time increase in atrial end-diastolic area (EDA) through saline injections (3 μL/min for 30 s). As shown in Figure 6A, each injection led to a significant increase in EDA, with values returning to baseline during the rest periods between injections. The average increase in EDA was 20.5% ± 2.0% (Figure 7).
Figures 6B and 6C show that the increase in preload had a minimal effect on heart rate (HR) during the first two loading periods, with a slight decrease in HR during the third injection. Stroke area (SA) increased significantly after each injection, with an average 60.1% ± 8.5% increase in SA (Figure 7). Importantly, the increase in cardiac output (COA) was driven solely by the increase in SA, as no significant change in HR was observed. The COA increased by 58.1% ± 8.8% (Figure 7), highlighting the role of mechanical modulation in enhancing atrial output without substantial changes in heart rate.
These findings suggest that, during early developmental stages (48 hpf), acute atrial preload increases primarily lead to mechanical changes in stroke area and cardiac output, with minimal contribution from electrical responses, indicating that mechanisms like MEC may not yet be active at this stage.
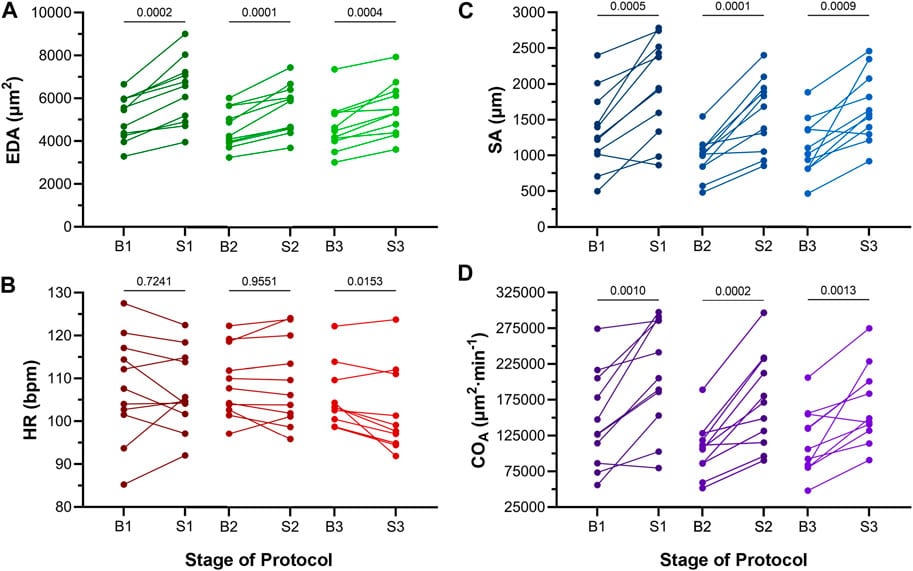
Figure 6: Effects of atrial dilation on functional parameters in 48 hpf zebrafish larvae. (A) End-diastolic area (EDA), (B) heart rate (HR), (C) stroke area (SA), and (D) area cardiac output (COA = HR × SA) immediately before (B1-B3) and at the end of load application (S1-S3). 1
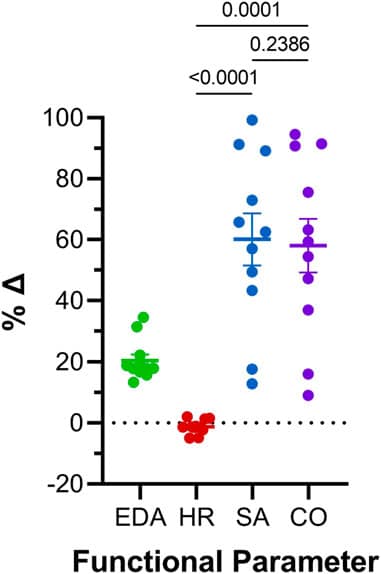
Figure 7: Comparison of effects of atrial preload application on functional parameters in 48 hpf zebrafish larvae.1
Conclusion
In this paper, researchers from the Cardiac, Autoregulation & Arrhythmias Laboratory at Dalhousie University presented a novel methodology, utilizing Fluigent’s advanced pressure based flow control technology, enabling precise modulation of mechanical load in the zebrafish heart model. This approach provides new insights into the role of MEC and MMC during cardiac development, allowing for real-time imaging of functional effects. It offers a powerful tool for future studies exploring the molecular mechanisms underlying these responses, the impact on cardiac diseases, and potential therapeutic strategies, particularly in congenital heart defects.
References
1. Baillie, J. S., Gendernalik, A., Garrity, D. M., Bark, D. & Quinn, T. A. The in vivo study of cardiac mechano-electric and mechano-mechanical coupling during heart development in zebrafish. Front. Physiol. 14, (2023).
2. Quinn, T. A. & Kohl, P. Rabbit models of cardiac mechano-electric and mechano-mechanical coupling. Prog. Biophys. Mol. Biol. 121, 110–122 (2016).
3. Quinn, T. A. & Kohl, P. Mechano-sensitivity of cardiac pacemaker function: Pathophysiological relevance, experimental implications, and conceptual integration with other mechanisms of rhythmicity. Prog. Biophys. Mol. Biol. 110, 257–268 (2012).
4. Rose, R. A. OBSOLETE: Cellular Sinoatrial Node and Atrioventricular Node Activity in the Heart. in Reference Module in Biomedical Sciences B9780128012383997599 (Elsevier, 2018). doi:10.1016/B978-0-12-801238-3.99759-9.
5. Katz, A. M. Ernest Henry Starling, His Predecessors, and the “Law of the Heart”. Circulation 106, 2986–2992 (2002).
6. Reed, A., Kohl, P. & Peyronnet, R. Molecular candidates for cardiac stretch-activated ion channels. Glob. Cardiol. Sci. Pract. 2014, 19 (2014).
7. Cairelli, A. G. et al. Role of tissue biomechanics in the formation and function of myocardial trabeculae in zebrafish embryos. J. Physiol. n/a, (2024).
8. Rödel, C. J. & Abdelilah-Seyfried, S. A zebrafish toolbox for biomechanical signaling in cardiovascular development and disease. Curr. Opin. Hematol. 28, 198–207 (2021).
9. Rafferty, S. A. & Quinn, T. A. A beginner’s guide to understanding and implementing the genetic modification of zebrafish. Prog. Biophys. Mol. Biol. 138, 3–19 (2018).
10. Bournele, D. & Beis, D. Zebrafish models of cardiovascular disease. Heart Fail. Rev. 21, 803–813 (2016).
11. Gendernalik, A., Zebhi, B., Ahuja, N., Garrity, D. & Bark, D. In Vivo Pressurization of the Zebrafish Embryonic Heart as a Tool to Characterize Tissue Properties During Development. Ann. Biomed. Eng. 49, 834–845 (2021).
Related products
Expertise & Resources
-
Microfluidics Case Studies UV-C and microfluidics for biofilm studies as a new experimentation method Read more
-
Microfluidic Application Notes What is the best method for Microencapsulation of Bacteria and Yeast in Small Double Emulsions? Read more
-
Microfluidic Application Notes Microbiome culture in droplet using dsurf surfactant Read more