University of Rochester: A tissue chip platform for real-time sensing of secreted inflammatory markers using ARIA
The University of Rochester, established in 1850 and located in New York State, offers extensive programs of study and research in various fields, including the life sciences. The university is home to several advanced centers and institutes dedicated to improving society through research and innovation.
The Department of Biomedical Engineering is renowned for its interdisciplinary research aimed at enhancing human health. The department’s research encompasses a broad range of areas, such as medical imaging, biomaterials, tissue engineering, and neural engineering. Researchers in the department collaborate with partners to develop novel therapies and medical technologies to benefit patient outcomes.
Through a partnership with the University of Rochester’s Department of Dermatology and the medical diagnostics company QuidelOrtho, a cutting-edge technology that utilizes a state-of-the-art microfluidic system in the context of a novel tissue chip platform was developed. This technology enables the real-time sensing of specific biomolecules to facilitate the search for treatments for inflammatory diseases, including lung infections.
In collaboration with the University of Rochester’s Biomedical Engineering department
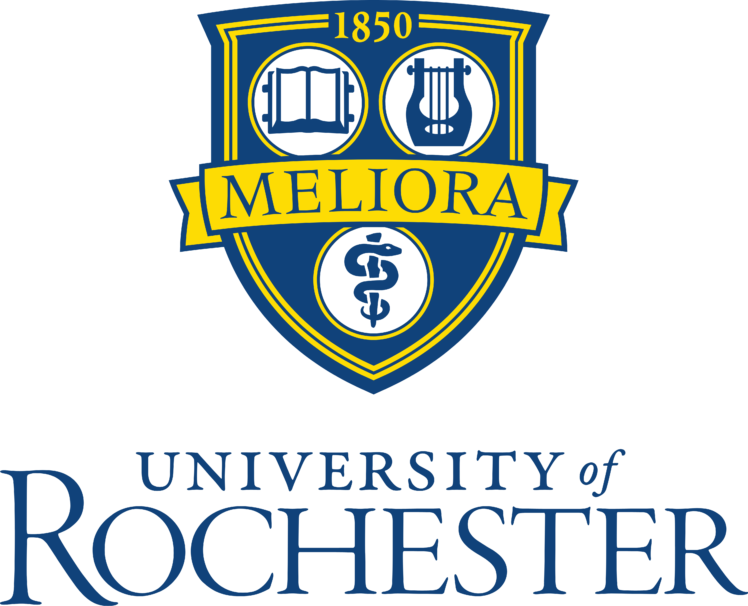
In collaboration with the University of Rochester’s Biomedical Engineering department
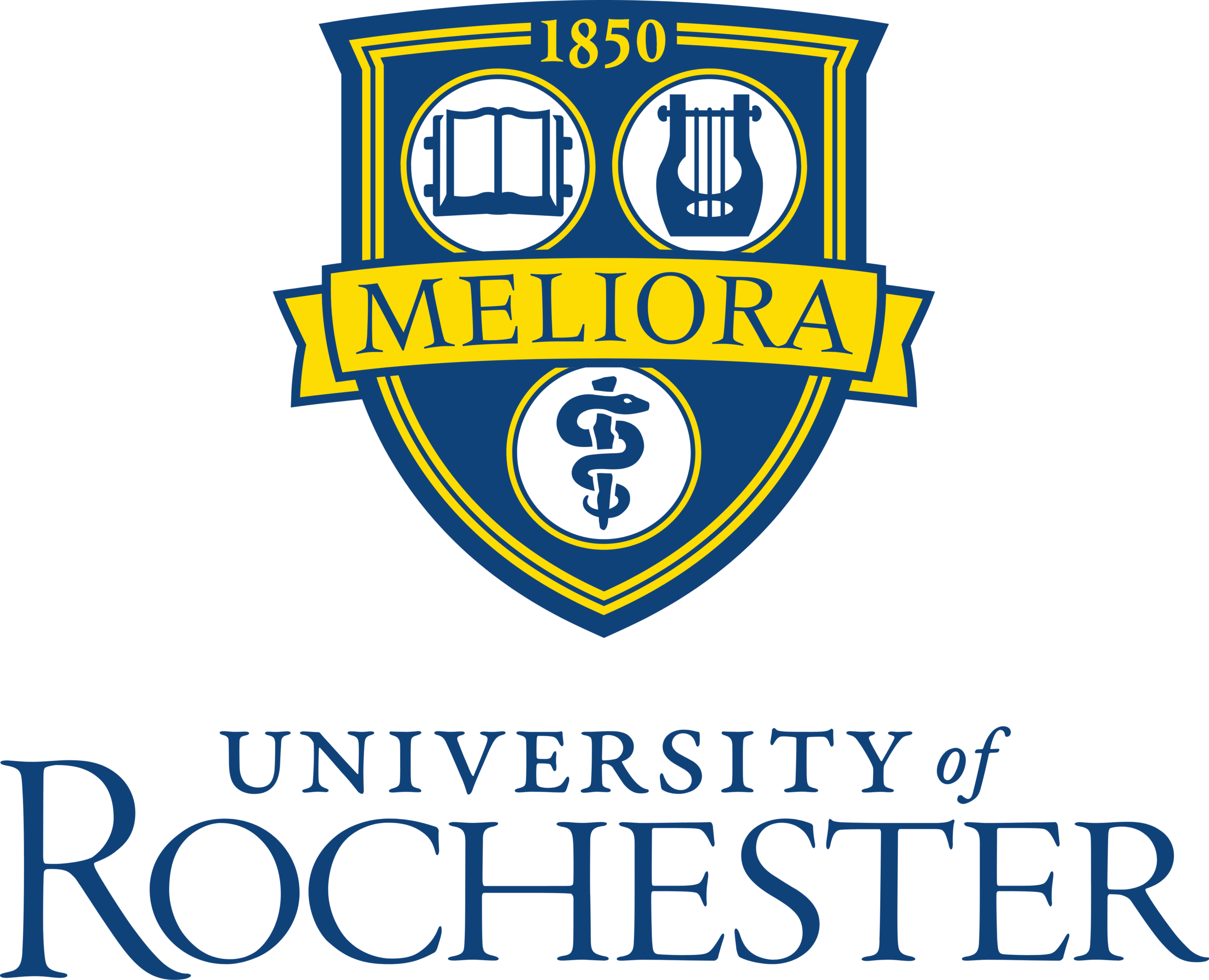
Testimonial
“While developing a new type of tissue chip that integrates real-time biosensing capabilities, I needed a microfluidic system that could deliver varied samples at precise flow rates. The Aria’s intuitive interface and automation software make it very easy to use for long-term experiments with many steps, and the internal fluidic routing conveniently avoids interfering with our already-complicated optical experimental setup. Also, the flow rate readout and feedback make it easy to control pressure inside our microfluidic devices and prevent bubbles, which is very important for our cell-based experiments. Fluigent was incredibly helpful in supporting us as we introduced their equipment into our lab and continues to provide excellent support when we (rarely) need it.”
John Cognetti, University of Rochester
Why develop a photonic biosensor-integrated tissue chip model?
An alternative to animal studies for drug discovery
Although animal studies are currently the preferred option for evaluating the safety, efficacy, pharmacokinetics, and toxicity of potential treatments, their results are often difficult to correlate with human outcomes due to physiological differences and genetic variations between species. Additionally, animal studies can be costly, require a large workforce, and raise ethical concerns due to the need for a significant number of animals. Human clinical studies also face challenges due to the heterogeneity of individuals and the complexity of the studies. Therefore, microfluidic models in a chip format may offer an efficient alternative to animal studies, providing a reliable, cost-effective, and ethical approach for drug discovery.
Advancements in In Vitro Models: Tissue Chips Mimicking Human Organs and Tissues
In vitro models are an alternative to animal models that can overcome the problem of heterogeneity between humans and animals and can also reduce costs and avoid ethical issues. However, previous in vitro models were limited in complexity and could not reflect physiological relevance of in vivo tissues or organs. To address this issue, microfluidic technologies, such as the tissue chip platform developed by the Biomedical Engineering department at the University of Rochester, were developed. This technology incorporates a microfluidic delivery system capable of mimicking the complexityof human organs and tissues, including 3D architectures.[1] These chips provide a physiologically relevant level of tissue organization, geometry, soluble gradients, and mechanical stimulation in an accurate and regulated manner. [2] This technology represents a significant advancement in drug discovery and development, as it allows for the development of more predictive methods for assessing the toxicity and efficacy of new compounds and therapeutics, as well as disease modeling and characterization. [1] Moreover, the integration of sensors into these microfluidic chips enables high throughput analysis.
Benefits of using a photonic biosensor integrated into the chip
The primary objective of this study is the real-time sensing of specific biomolecules. Detecting cytokine secretion by epithelial cells in real-time is a crucial goal for tissue chip technologies, as cytokines play a vital role in various inflammatory diseases such as lung infections and can act as useful biomarkers. Unfortunately, few techniques have been developed to determine the cellular response to a stimulus in real time, and the few that exist may utilize fluorescent tags that can disturb the system or lack specificity in their biosensing capabilities. [4] As a result, researchers from the Department of Biomedical Engineering at the University of Rochester have taken up this challenge and developed a new platform based on tissue chip technology. The purpose of this tissue chip platform is to measure the secretion kinetics and concentrations of analytes in close proximity to the lung epithelial cells, which will aid in better understanding the disease dynamics. To maximize the sensing performance, a photonic biosensor has been integrated into the chip at extreme proximity to the cells, which represents an innovative, label-free, and highly sensitive solution.
Using the ARIA in the tissue chip platform for real-time sensing of inflammatory markers
Description of the tissue chip platform
The University of Rochester has developed a new, innovative platform with the goal of improving existing tissue chip models and creating a device capable of sensing the secretions related to the inflammation of lung epithelial cells in real time. This platform consists of various components listed below:
- A microfluidic tissue chip: This microfluidic device includes two microfluidic channels separated by a thin nanoporous membrane. The upper channel is used for sample supply, while the lower channel is sealed and in direct contact with the biosensor, which collects the inflammatory markers secreted by the cells. The lowest layer of the chip is equipped with a photonic biosensor that can capture certain resonance wavelengths that are modified by the refractive index of entities in close proximity, thanks to 14 rings functionalized according to the targeted analyte. Thus, in the presence of cytokines around the rings, a shift in the resonance wavelengths of the rings towards the red end of the spectrum may be observed in a manner consistent enough to be quantified according to the concentration of cytokine in the medium. The membrane is made of a nanoporous silicon nitride membrane (NPN), optically clear, composed of four slots and serves as a substrate for the cell culture. The cells will be able to adhere to it and create tight junctions in the form of a monolayer network.
- Fluid delivery: To supply the tissue chip device continuously during the experiment, our automated sequential injection system, the ARIA, is used upstream of the chip. It is controlled by a pressure-driven fluid pump and can be pre-loaded with up to 10 different samples. A switch function allows for seamless transition between samples, and a bubble trap prevents air from disrupting the cells in the device.
- Optical configuration: An optical set-up is also used to capture the resonance shifts transmitted by the sensors in real time. This includes a tunable laser source, a fiber array aligned to the chip, and visible IR cameras.
- Data analysis: Finally, a custom python program is used to compute the resonance shifts transmitted by the sensors and captured by the optical set-up, allowing the experimenter to access this data via an interface. The interface allows users to select the peaks and categorize them according to the nature of the sensor ring (control or capture) and thus the relative shift is calculated depending only on the specific capture of the analytes.
The tissue chip platform is placed in an aluminum stage fitted with a thermoelectric coupler and a thermistor to maintain the device at 37°C.
Course of the experiment
Human bronchial epithelial cells (16HBE line) are used in the tissue chip model due to their ability to form tight monolayers in vitro, attributed to the presence of a robust network of tight junctions. These cells are also known to secrete cytokines in response to stimulation with bacterial endotoxin lipopolysaccharide (LPS). A complete or nearly complete monolayer of cells is seeded into the device, covering the four membrane slots. The Aria reservoirs are preloaded with the necessary solutions (media, LPS, controls) and the platform is maintained at 37°C. During sensing experiments, the two channels are disconnected from each other. The bottom channel is sealed with binder clips to capture analytes produced during passive cell diffusion on the photonic biosensor, while the inlet of the top channel is connected to the outlet tubing of ARIA. The medium is diffused at a continuous rate of 30 µL/min through the top channel during 30min, followed by the LPS at 100 ng/mL for two hours. By aligning the chip to the fiber array, spectral measurement can begin immediately, and data is acquired in real-time.
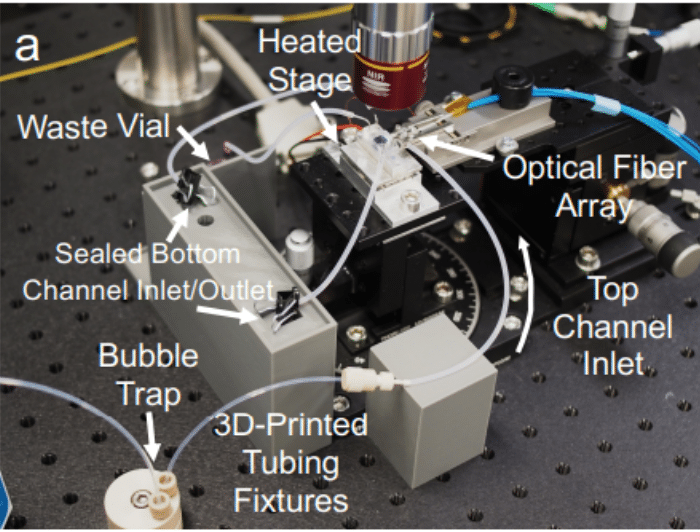
Figure 3: Set-up of the platform.
Partial results
Control tests
Initially, a single channel microfluidic device was used to calibrate the sensors with four analytes. The pro-inflammatory cytokines IL-1β, IL-6, and IL-8 were tested, along with the C-reactive protein (CRP), which acted as a negative control for cell stimulation (not secreted by the 16HBE), and a positive control for the sensor (120kDa pentamer easily detected by the photonic biosensor; added exogenously at the end of the experiment). The four tests confirmed that the relative shift in pm is directly proportional to the analyte concentration. Next, a second test was conducted to ensure that the 16HBE cells behaved as expected and formed tight junctions. This was proven using the junction marker ZO-1 after four days of cell culture in the device. Finally, a control was performed to prove that analytes originating from the membrane can indeed reach the membrane in a short time. The assay was conducted on the analytes IL-1β, IL-6, and IL-8 in a cell-free chip, and relatively similar shifts were observed after 5-10 minutes after the entry of the different analytes into the top channel. This validated the possibility of acquiring time-resolved data of the cellular response to a stimulus added to the top channel.
The tests confirmed the proper functioning of the different key components of the tissue chip device. The next step involves testing the platform under experimental conditions.
Sensing of cellular secretion partial results
The media flowed via the ARIA for 30 minutes on the complete platform described above, followed by the injection of LPS at 100ng/mL for two hours. One hour after the injection of LPS, a sensor response was observed, which resulted in an increase in the relative shift for interleukin IL-1β and IL-6 secreted by the lung epithelial cells. Equilibrium was reached between 90-120 minutes. This demonstrates that interleukins can diffuse to the biosensor located at the bottom of the tissue chip. The response was shown to be specific to the cytokines secreted by HBEs by analyzing the curve in Figure 4c, which shows the lack of variation of the relative shift as a function of the CRP concentration. An additional test was performed using a membrane without cells on some of its slots, revealing that the shift is less strong for the sensors farther away from cells.
In summary, these experiments have demonstrated that the photonic tissue chip platform can detect physiologically relevant concentrations of analyte and report on the quantity of cytokines secreted by inflamed epithelial cells in real time following LPS stimulation. Additional tests have also shown that these sensors can provide data with both spatial and temporal resolutions.
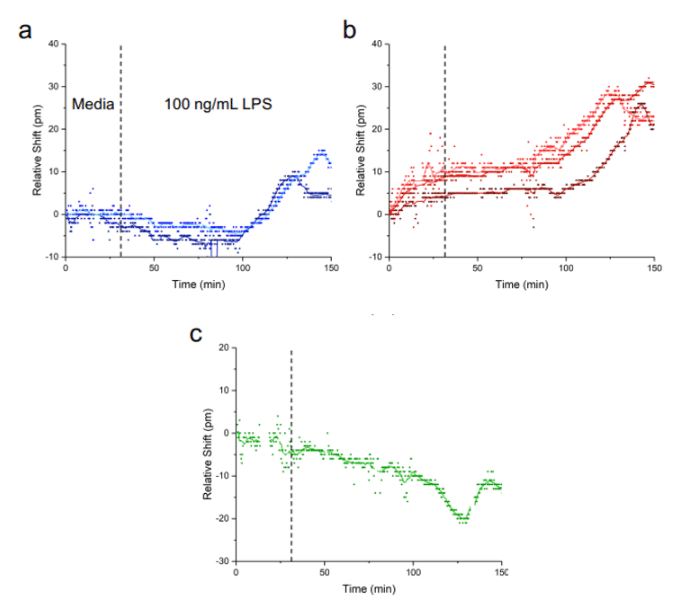
References
[1] Low LA, Tagle DA. Tissue chips – innovative tools for drug development and disease modeling. Lab Chip. 2017;17(18):3026-3036. doi:10.1039/C7LC00462A
[2] Donoghue L, Nguyen KT, Graham C, Sethu P. Tissue Chips and Microphysiological Systems for Disease Modeling and Drug Testing. Micromachines 2021, Vol 12, Page 139. 2021;12(2):139. doi:10.3390/MI12020139
[3] Microfluidic white paper – A guide to Organs-on-Chips technology, Fluigent
[4] Morales AW, Zhang YS, Aleman J, et al. Label-free detection of protein molecules secreted from an organ-on-a-chip model for drug toxicity assays. In: Frontiers in Biological Detection: From Nanosensors to Systems VIII. Vol 9725. SPIE; 2016:972508. doi:10.1117/12.2212971