CNRS/UTC: study of a liver-on-a-chip model
Founded in 1972, the Compiègne University of Technology (UTC) is a research and educational institute with the aim of positioning technology at the center of interdisciplinary research projects. Among the 3 main research axes, the “technologies for healthcare” field is of particular importance as bringing technologies to biomedical research allows for a better understanding of the ways to treat pathologies, improved diagnostic methods,developnew in-vitro models for drug testing, and more..
UTC, in partnership with various academic and industrial partners, relies on 8 research units. Among them is the Biomechanics and Bioengineering CNRS research center, where the interactions between fluids and biological structures are heavily studied. In this field of research, microfluidic systems are continuously developed, as they are perfectly suited to make miniaturized, robust, and controllable systems involving fluid handling and biological functions, such as human liver-on-a-chip models. Learn more about UTC.
Testimonial
“FLUIGENT pressure controllers were used before my arrival at BMBI laboratories to control the fluid circulation when studying fluid structure interactions. The goal of these experiments was to measure the deformation of soft flowing object in order to evaluate their mechanical properties. An accurate knowledge of the flow strength is key to the success of this approach.
I was the first in the team to use pressure control for cell culture experiments. I compared the behavior of cells in dynamic culture in biochips combined with pressure controllers and peristaltic pumps. Thanks to FLUIGENT MFCS systems, I could monitor the pressure applied at the inlet reservoir and demonstrate that it is correlated with the number of cells inside the biochip. This kind of information cannot be obtained when a peristaltic pump is used. Cell growth inside the chip may eventually lead to the clogging of the flow circulation.
This clogging can be anticipated when pressure-controlling the fluid circulation in the system, while when using a peristaltic pump, it might remain undetected until the detachment of the chip. In addition, the use of pressure-controlled flow is crucial when working with pressure sensitive cells such as endothelial cells which require a precise monitoring of the pressure variations. During my work at BMBI laboratory, I saw an expansion of the use of FLUIGENT pressure controllers. Thanks to their simplicity, user friendly interface and their accuracy, different applications are being explored.
New research projects are continuously being launched and the use of FLUIGENT pressure controllers to induce the flow is strongly proposed thank to the non-invasive feedback that such technology confer.”
Taha Messelmani, PHD student at Université de Technologie de Compiègne | UTC · BioMécanique et BioIngénierie (BMBI)
Why develop a microfluidic model for liver tissue culture?
Limitation of animal models for drug discovery
Drug development is a long process that can take up to 15 years [1] and include several requirements. Some drug development processes such as animal testing, are subject to strong debates like the ethical relevance of involving animals in the process. In addition, the final product can fail to receive authorization for commercialization due to toxicity or insufficient efficacy [2]. Animal models not only are being strongly discussed due to ethical questions, but they also show limitations for drug development as there are many differences between animal and human biology [3].
Advantages of microfluidic organ on–a-chip models
To overcome these limitations, in-vitro models are being developed to better mimic the human environment and hence accurately determine the human response to drugs. In particular, organ-on-a-chip models are of special interest, as they allow the culture tissue to be studied under dynamic and regulated conditions, reproducing physiological shear-stress with controlled molecule concentrations and3D structures like the in-vivo.
UTC developed an in-vitro liver-on-a-chip model, one of the most promising organ-on-a-chip technologies for drug screening and biological assays, in which they investigate the growth of hepatic cells under dynamic conditions in a microfluidic chip (the biochip) composed of a chamber filled with a 3D hydroscaffold structure. In these conditions, the cells grow and gather to form spheroids, which are not observed in conventional 2D static cultures. Figure 1 summarizes the experiment and illustrates its main results: the self-organization of cells into spheroids and the functionality of liver cells are assessed by their level of secretion of urea and albumin. The organ functions of this human liver-on-a-chip model were found to be improved as compared to traditional culture methods, indicating the viability of the in-vitro model.
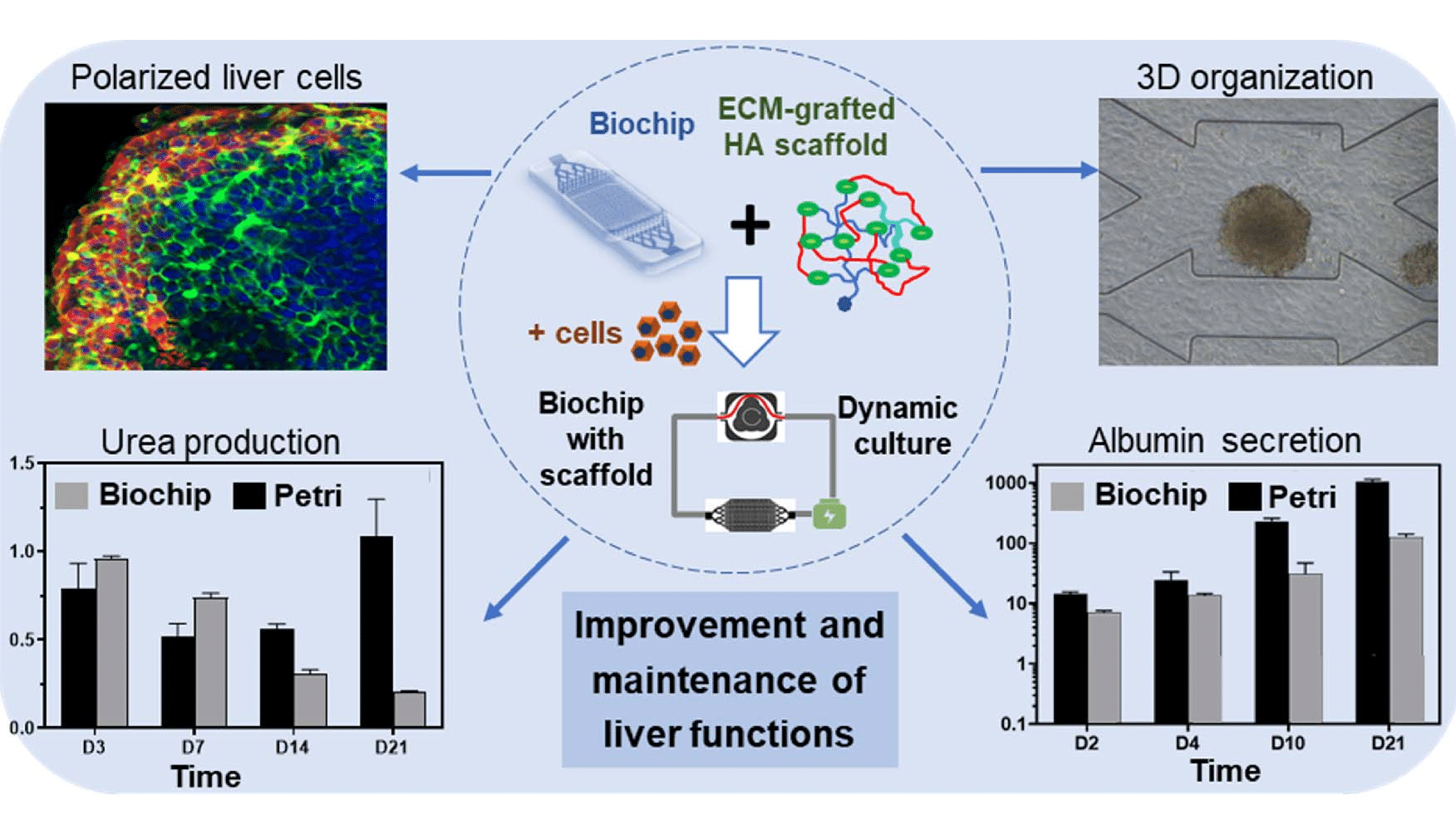
Figure 1: Graphical abstract of the liver-on-a-chip model
Use of Fluigent products for characterizing the microfluidic culture of liver tissue
In this study, a 3D hydroscaffold composed of hyaluronic acid was built to embed the cells and provide them with a suitable growth environment. Then, the cells are grown in this hydroscaffold, and due to medium providing shear-stress conditions and a 3D structure, they tend to form spheroids that increase in size over time (fig 2).
For the cells to grow efficiently and form a viable liver-on-a-chip model, the chip is constantly perfused with a culture medium. However, the 3D hydroscaffold and cell proliferation can have an impact on the ability of liquid to flow through the system due to potential clogging.
To monitor this clogging effect, a microfluidic setup consisting of Fluigent pressure-based flow controllers, MCFS-Ex and Flow units M, was created to not only impose the desired flow rate but also measure the pressure level in the chip at several timepoints (fig 3). The flow controller was connected to both the inlet and the outlet of the microfluidic chip, allowing the measurement of the pressure at each side of the liver-on-a-chip model. The flow unit was placed on the fluidic path to ensure a constant flowrate of 10µL/min, and the pressure difference was determined. An increase of pressure difference would mean clogging appeared in the system.
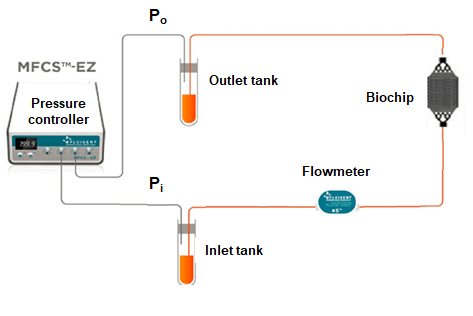
Figure 3: Fluigent setup used for pressure measurement
Partial results
The first step was to investigate the clogging effect with hydroscaffold in the chip. To do this, the pressure level was measured on the chip with and without the integrated hydroscaffold and compared between the 2 conditions. In Figure 4, we see that the pressure values are similar between the 2 conditions for various tested flowrates, demonstrating no clogging due to the presence of the hydroscaffold in the human liver-on-a-chip model.
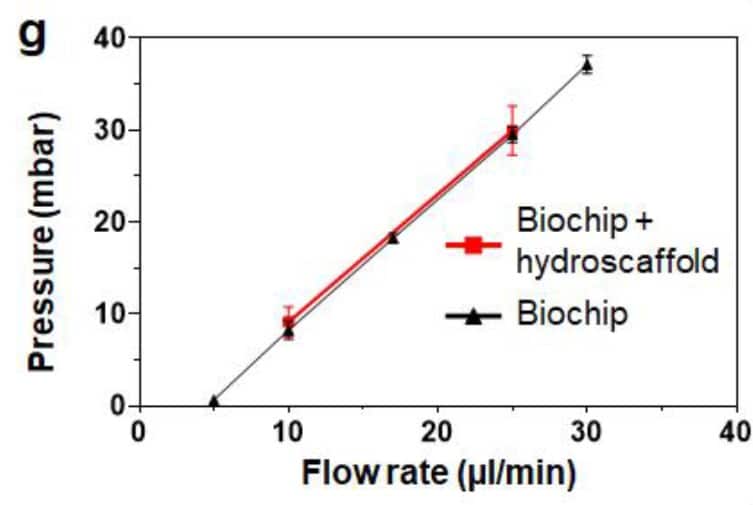
Figure 4: Pressure comparison between the empty biochip and the biochip filled with 3D hydroscaffold for various flow-rates
After cells were seeded in the biochip filled with the 3D hydroscaffold, the pressure difference was measured at 10µL/min at several timepoints (fig 5). During days 0 to 11, the pressure values were found stable, around 60mbar, with spheroid growing up to 450µm diameter. Despite the increase in cell numbers, the hydraulic resistance remains stable, and the medium can circulate between the spheroids. From day 14 on, a strong proliferation was observed (fig 2), and spheroids began to form large aggregates, occupying most of the biochip. Eventually, the aggregates caused clogging at day 21, demonstrated by the pressure rise seen in figure 5.C. The pressure then goes back to normal when the aggregate is flushed away. This pressure increase, up to 1.5 bar, caused chip leakage and experimental failure. The chip failure gave information on the time of viability of the liver-on-a-chip model.
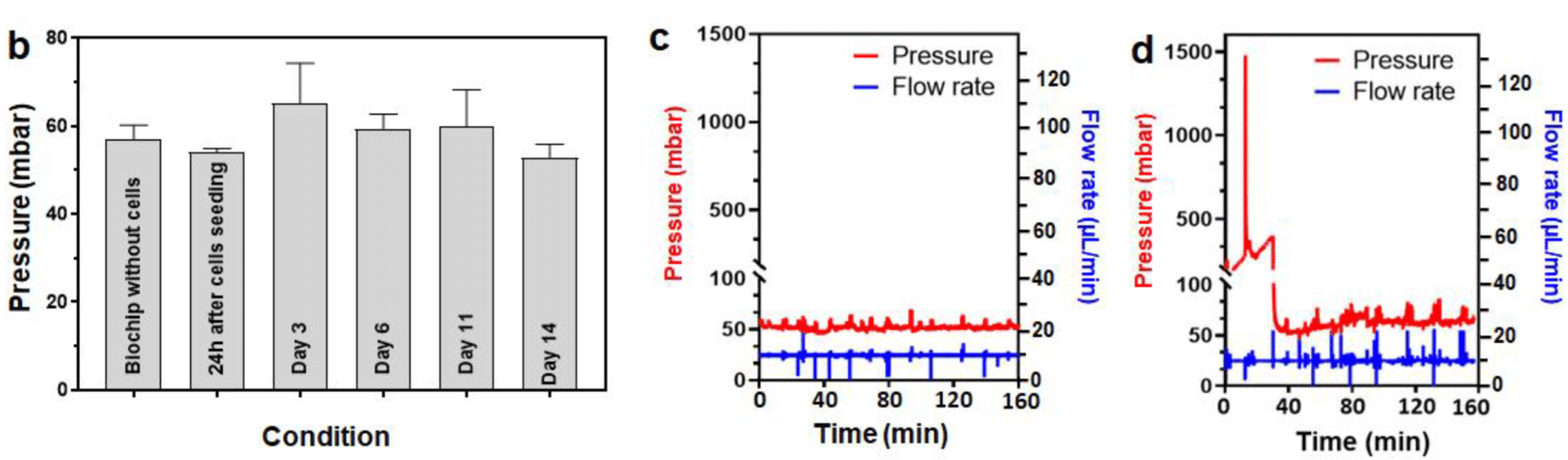
Figure 5: Pressure evolution during the 14 first days of culture (a), and pressure measurement inside the chip at days 14 and 21 (b,c)
References
[1] Hughes, J.P.; Rees, S.; Kalindjian, S.B.; Philpott, K.L. Principles of early drug discovery. Br. J. Pharmacol. 2011, 162, 1239–1249. [Google Scholar] [CrossRef]
[2] Freyer, N.; Knöspel, F.; Strahl, N.; Amini, L.; Schrade, P.; Bachmann, S.; Damm, G.; Seehofer, D.; Jacobs, F.; Monshouwer, M.; et al. Hepatic differentiation of human induced pluripotent stem cells in a perfused three-dimensional multicompartment bioreactor. Biores. Open Access 2016, 5, 235–248. [Google Scholar] [CrossRef]
[3] Merlier, F.; Jellali, R.; Leclerc, E. Online hepatic rat metabolism by coupling liver biochip and mass spectrometry. Analyst 2017, 142, 3747–3757. [Google Scholar] [CrossRef]
Expertises & Resources
-
Microfluidics case studies A microfluidic Artery-on-a-Chip using Fluigent’s Microfluidic Flow Control System, the MFCS Read more
-
Microfluidics case studies Creating a Microfluidic Cancer-on-Chip Platform using Fluigent’s High Throughput Cell Perfusion Pack Read more
-
Microfluidic Application Notes Automating Neuronal Cell Immunofluorescence in Microfluidic Chips Read more
-
Expert Reviews: Basics of Microfluidics Microfluidic pressure control for organ-on-a-chip applications: A comprehensive guide Read more
-
Microfluidics case studies University of Rochester: A tissue chip platform for real-time sensing of secreted inflammatory markers using ARIA Read more
-
Microfluidics White Papers Microfluidic white paper – A review of Organ on Chip Technology Read more
-
Expert Reviews: Basics of Microfluidics How to choose a microfluidic chip Read more
-
Microfluidic Application Notes Peristaltic Pump vs Pressure-Based Microfluidic Flow Control for Organ on Chip applications Read more