Creating kidney organoids‑vasculature interaction model using Fluigent’s Flow-EZ
Kidney organoids derived from human induced pluripotent stem cells (iPSCs) are essential for studying kidney development and disease. However, their lack of vascularization often leads to insufficient oxygen and nutrient supply. Traditional methods using animal models result in vasculature mainly from the host tissue. The goal of this study is to create kidney organoids in vitro model with fully human-cell-derived vascularization.
Using their microfluidic chip system, Menéndez et al. cultured kidney organoids displaying nephron structures and increased maturation of endothelial populations. Human umbilical vein endothelial cells (HUVECs) within the chip's channels migrated and proliferated into the organoid tissue, forming vessel-like structures with open lumens.
This study is the first to achieve vascularization of kidney organoids in HUVEC co-culture conditions using a microfluidic organ-on-chip. This model offers valuable insights into kidney organoid vascularization and is a promising tool for studying kidney development and drug testing.
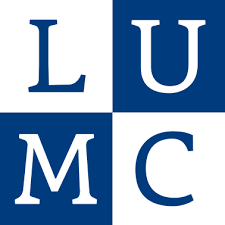
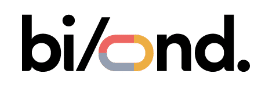
Kidney Organoids for mimicking human fetal kidneys
Kidney organoids are three-dimensional structures resembling human fetal kidneys (1), derived from two embryological niches that give rise to the kidney: the ureteric bud and metanephric mesenchyme. These organoids develop nephron structures, including glomeruli with podocytes, proximal tubules, and distal tubules. Due to their similarity to native tissue, these structures are valuable for understanding kidney development and studying diseases (2). However, challenges such as limited culturing times and lack of vascularization result in insufficient oxygen and nutrient supply, leading to necrotic core formation and limiting their size and maturation.
Despite the presence of some endothelial cells (ECs) and high expression of vascular endothelial growth factor (VEGF) in kidney organoids, traditional methods fail to achieve perfusable endothelial tissue expansion and maturation. Organoids exhibit endothelial maturation markers like MCAM (CD146) and PECAM (CD31), crucial for vascular development. Previous studies have shown that MCAM+ cells are vital for kidney vasculature development.
Microfluidics technology for kidney organoids-on-a-chip
Current approaches to vascularize kidney organoids include implantation in animal models (3,5), but this results in vasculature derived from the host tissue. In vitro vascularization is a novel approach to overcome these issues. Fluidic shear stress (FSS) in a millifluidic bioreactor has shown promise in promoting vascular structure development by establishing a VEGF gradient.
In this study, authors introduce a novel method for vascularizing kidney organoids using the BIOND microfluidic organ-on-chip with perfusable channels. Organ-on-chip systems are valuable for developing vascular models due to their ability to compartmentalize and co-culture different cell types. Perfusable endothelial-coated channels, created using HUVECs, have successfully formed microvascular structures with lumens in vitro. The goal is to achieve endothelial ingrowth inside kidney organoids via co-culture with synthetic vessels in an organ-on-chip, creating an efficient model for organoid vascularization.
Materials and methods
Organ‑on‑chip microfluidic system
The authors used the BIOND organ-on-chip system (inCHIPit™ and comPLATE™) from BIOND Solutions B.V., Delft, The Netherlands. The inCHIPit™ (Figure 1) features a culture chamber connected to three 400 µm-wide channels through 4 µm-wide pores. To generate flow, the chips are placed on the comPLATE™, which is connected to Fluigent’s pressure pump FlowEZ that creates 800 mbar of pressure, circulating medium from the reservoir through the tubing and into the chip channels. This setup produces an estimated flow rate of 0.2 µL/min across the porous membrane. The system maintains a constant flow due to Fluigent’s flow unit, ensuring a continuous supply of fresh medium and stable shear stress. These perfusable channels enable to sustain organoid cultures under fluidic flow, a crucial factor for vasculature development.
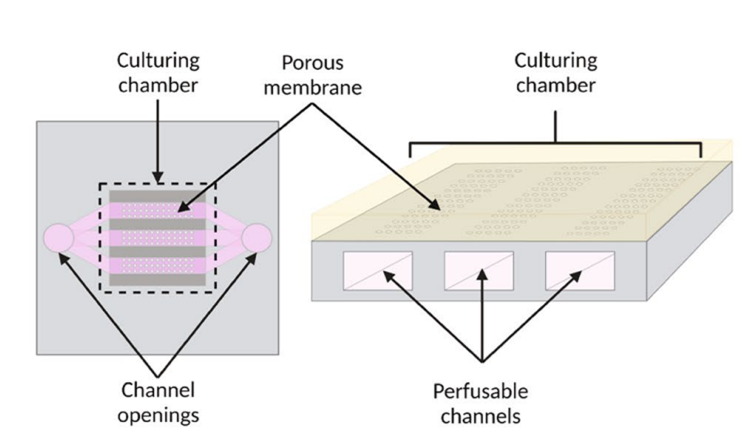
HUVEC seeding on chip channels
Human umbilical cord endothelial cells expressing GFP were cultured in the chip channels statically for 48 hours, with the medium replenished after 24 hours. After 48 hours, the chips were connected to a flow setup with a constant microfluidic flow rate of 3 µL/min for 48 hours before placing the kidney organoids in the top chamber. Confocal imaging and Fiji software were used for 3D rendering and analysis of HUVEC directionality. To assess directionality cells that showed an elongation and direction concurrent with the flow direction with a deviation not greater than 45° were considered as having acquired directionality (Figure 2).
FIgure 2: (A) fluorescence image of the chip’s three microfluidic channels after 48 h of static culture, showing GFP+ HUVECs forming a monolayer inside these channels. (B) Fluorescence image of a chip channel after 48 h of static culture+24 h of flow, showing HUVECs remained in the channels after exercising fluidic stress and adopted a directionality concurrent with the flow direction. (C) Detail images of the chip channels after 48 h of static culture+24 h of flow, in which the directionality acquired by the HUVECs can be observed (indicated with arrow). (D) 3D render of confocal stack of a chip channel lined with GFP+ HUVECs showing establishment of a 3D synthetic vessel presenting cells in all planes.
On chip culturing of kidney organoids
After 5 days of culture in the transwell system, kidney organoids were placed in the top well of the microfluidic chip system, with two organoids per chip. The culture medium used was suitable for co-culture conditions. The flow rate was maintained at 3 µL/min, and organoids were collected after 9 days for fixation and processing (Figure 3).
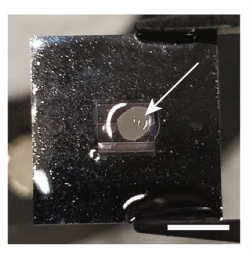
Figure 3: Kidney organoid on chip (indicated with arrow) at day 20 of the protocol before collection.
Partial results
Co‑culture of kidney organoids with endothelialized channels
Co-culturing kidney organoids with endothelialized channels promotes HUVEC migration into the organoid tissue and the formation of open lumen structures. To investigate this, the authors lined the chip channels with GFP+ HUVECs and cultured organoids above them for 9 days (Fig. 4A).
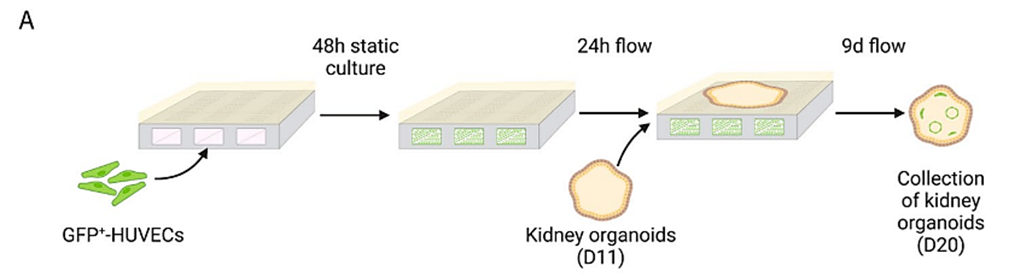
Figure 4: Schematic representation of the co-culture timeline of HUVECs and kidney organoids, indicating static culture, exercise of flow and addition and collection points of kidney organoids.
Upon co-culture with the channels endothelialized with GFP HUVECs, organoid tissue presented GFP+ HUVECs forming vascular structures that connected to native organoid ECs. (Fig. 5). These GFP+ structures also connected with the organoid’s endogenous ECs (GFP-) as confirmed by PECAM immunostaining. These results demonstrate that co-culture of kidney organoids and HUVECs on the chip system leads to endothelial cell infiltration and vascularization under microfluidic flow.
The authors present the first successful infiltration of HUVECs inside kidney organoids where they contribute to vascular development.
Conclusion
Kidney organoids derived from human iPSCs are a powerful model for studying kidney development and disease, but their lack of vascularization limits their maturation and longevity.
In this work, the authors employed a novel organ-on-chip system to initiate their vascularization. Their findings show that this system supports kidney organoid differentiation and enhances both the number and maturation of endogenous endothelial cells. By seeding HUVECs in the microfluidic channels of the chip, they created synthetic vessels.
When co-cultured with the organoids, HUVECs migrated through the porous membrane and formed vascular-like structures within the organoid tissue, presenting open lumens and connecting with endogenous endothelial cells.
This research marks the first steps towards in vitro vascularization of kidney organoids, offering significant potential for developmental studies and drug testing.
Expertises and resources
-
Microfluidics Case Studies A microfluidic Artery-on-a-Chip using Fluigent’s Microfluidic Flow Control System, the MFCS Read more
-
Microfluidics White Papers A review of Organ on Chip Technology – A White Paper Read more
-
Expert Reviews: Basics of Microfluidics Why Control Shear Stress in Cell Biology? Read more
-
Microfluidics White Papers An exploration of Microfluidic technology and fluid handling Read more
-
Expert Reviews: Basics of Microfluidics Microfluidics overview: History and Definition Read more
Related Products
References
- Takasato, M. et al. Kidney organoids from human iPS cells contain multiple lineages and model human nephrogenesis. Nature 536, 238 (2016).
- Little, M. H. & Combes, A. N. Kidney organoids: Accurate models or fortunate accidents. Genes Dev. 33, 1319–1345 (2019)
- Garreta, E. et al. Fine tuning the extracellular environment accelerates the derivation of kidney organoids from human pluripotent stem cells. Nat. Mater. 18, 397–405 (2019).
- Xinaris, C. et al. In vivo maturation of functional renal organoids formed from embryonic cell suspensions. J. Am. Soc. Nephrol. 23, 1857–1868 (2012).
- 14. van den Berg, C. W. et al. Renal subcapsular transplantation of PSC-derived kidney organoids induces neo-vasculogenesis and signifcant glomerular and tubular maturation in vivo. Stem Cell Rep. 10, 751–765 (2018).