CEA/CNRS: A flow cell for nanoscopic imaging in liquid
Nanoscope imaging in liquid refers to the use of specialized imaging techniques to visualize and analyze samples at the nanoscale level in a liquid environment. This is a powerful tool for studying biological, chemical, and physical phenomena in liquid environments, such as living cells or complex fluids. It enables the observation of samples in their native state, without altering the sample structure and properties.
This makes it possible to study dynamic processes and interactions in liquid environments. Using this technology and Fluigent microfluidic devices, the LIONS laboratory has developed a flow cell for nanoscopic imaging to analyze inorganic particles in water.
LIONS: Interdisciplinary Laboratory on Nanoscale and Supramolecular Organization
The flow cell for nanoscopic imaging was developed by the Interdisciplinary Laboratory on Nanoscale and Supramolecular Organization (LIONS), one of the 7 laboratories of NIMBE (Nanoscience and Innovation for Materials, Biomedicine and Energy), a CEA-CNRS joint research project belonging to the University of Paris-Saclay, France. NIMBE’s research activity is focused on the design, processing, and analysis of matter from micron scale to nanoscale, and on the understanding of physicochemical mechanisms and their synergies. LIONS has 15 permanent members (with a 16th to be added soon) and 12 PhD and master’s students whose activities focus on the understanding and utilization of self-assembly for creating useful and safe supramolecular architectures. They have published over 500 research papers in some of the world’s most prestigious academic journals, and have filed more than 10 patents since 2006. Today, they focus on 3 main research topics: rational synthesis of nano-objects (nucleation growth, biomineralization …), reactivity at the nanoscale and under confinement (catalysis, radiolysis, energy storage…), and nano/bio interactions (nanomedicine, nanotoxicity, diagnostics…).
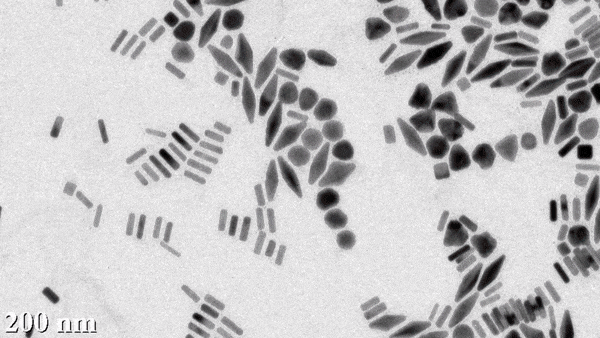
To support these research topics, LIONS has developed its own specific tools (homemade SAXS, irradiation devices, 3D printed specific sample environments, lab on a chip, etc.). Among those tools, microfluidics offers an ideal environment for controlling the synthesis of nano-objects, such as inorganic nanoparticles, and for characterizing the nucleation pathway. Designed to be efficiently coupled to analytical techniques, it should lead us to a completely renewed understanding of the very early stages of crystallization.
In a paper published in July 2020 in collaboration with the ENS Paris and the SOLEIL synchrotron, LIONS researchers present and fully characterize a flow cell for nanoscopic imaging.1 Its use as a routine sample environment for soft X-ray spectromicroscopy is demonstrated through the spectral analysis of inorganic particles in water.
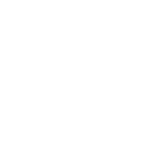
“The stepwise variation of the inlet pressure resulted in similarly stepwise changes in the flow rate values, with no detectable transients. This result was attributed to the quality of the pressure controller […]”
Corinne Chevallard – NIMBE/LIONS, CEA-CNRS
A pressure-actuated flow cell for nanoscopic imaging using soft x-ray spectromicroscopy in liquid media
Introduction
Transmission spectromicroscopes relying on electron or soft X-ray beams are traditionally employed to image and characterize samples at the micro- and nanoscales in fields like materials science, geology, and biology. As these approaches are subject to experimental constraints such as micrometer sample thickness and a vacuum environment, there is a huge interest in performing in situ studies. Today this is mainly done by using closed flow cells with an electron-transparent window (usually silicon nitride membranes) to tightly isolate the liquid sample from the microscope vacuum.
Today, many in situ TEM studies exist, but few of them have utilized closed cells for in situ scanning transmission X-ray microscopy (STXM). The latter is complementary to STEM, as it can provide both structural and chemical information on larger objects, like biological cells, with fewer artefacts and a suitable spatial resolution.
Using Fluigent products to develop a flow cell for nano-scale imaging
To fill this gap, the LIONS laboratory and collaborators have developed a dedicated sample environment for STXM. They worked on the design, hydrodynamic characterization, and complete validation of a new flow cell for nanoscopic imaging using STXM in liquid environments. The central element of this system is a flow cell that channels the liquid between two silicon chips supporting the SiN windows. More specifically, these two chips form a silicon chamber a few microns thick, and are arranged in a holder with micromachined fluidic access ports (Fig. 2). Fluid is actuated using a Fluigent MFCS-EZ pressure controller, which applies a controlled gas pressure on top of the solution to be injected (Fig. 1b). Fluigent M-switch and 2-switch valves are used to select the working fluid and to choose the fluidic pathway, either through or bypassing the cell.
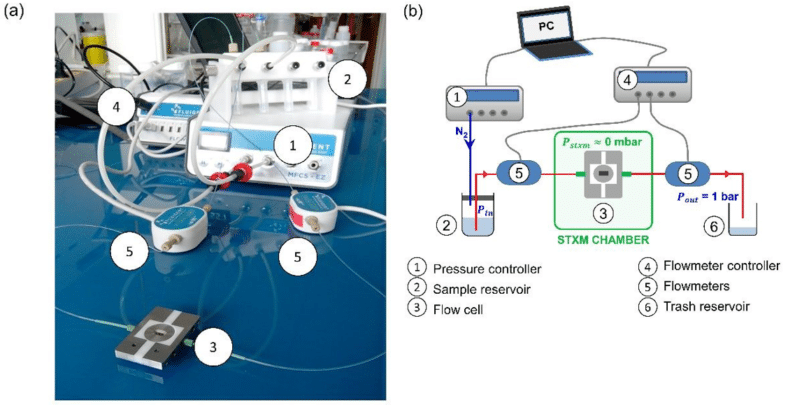
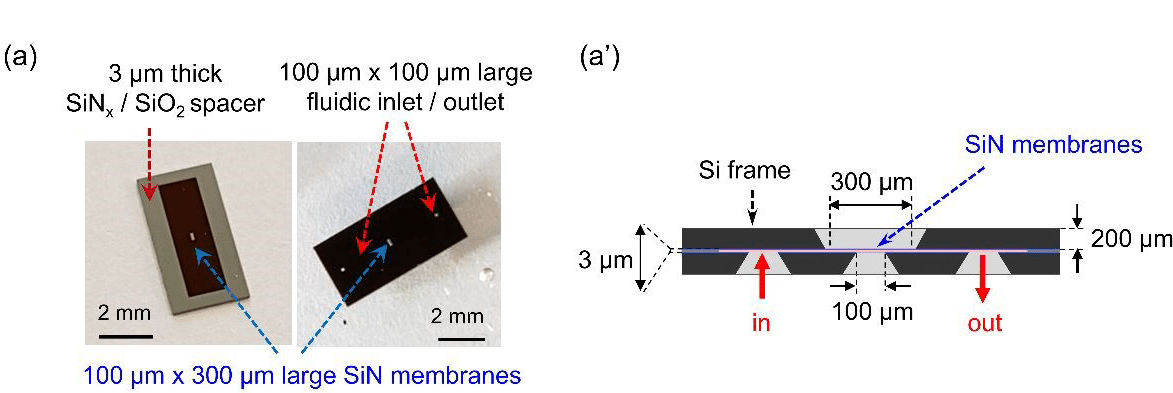
To fill this gap, the LIONS laboratory and collaborators have developed a dedicated sample environment for STXM. They worked on the design, the hydrodynamic characterization, and the full validation of a new fluidic system allowing imaging by STXM in liquid environment. The central element of this system is a flow cell that channels the liquid between two silicon chips supporting the SiN windows. More specifically, these two chips form a silicon chamber, a few microns thick, and are arranged in a holder with micromachined fluidic access ports (Fig. 2). Fluid is actuated using a Fluigent pressure controller MFCS-EZ, which applies a controlled gas pressure on top of the solution to be injected (Fig. 1b). Fluigent M-switch and 2-switch valves are respectively used to select the working fluid and to choose the fluidic pathway, either through or bypassing the cell.
Results
Response of the flow cell for nanoimaging to fluidic actuation
The authors varied the applied pressure difference, ΔP, while recording the flow rate both upstream and downstream of the flow cell using two Fluigent flowmeters. The stepwise variation of the inlet pressure resulted in similarly stepwise changes in the flow rate values, with no detectable transients (Fig. 3).
This result was attributed to the quality of the pressure controller and to the rigidity of both the tubes and the flow cell. Identical values recorded by the two flowmeters confirmed that there were no leaks in the microfluidic system.
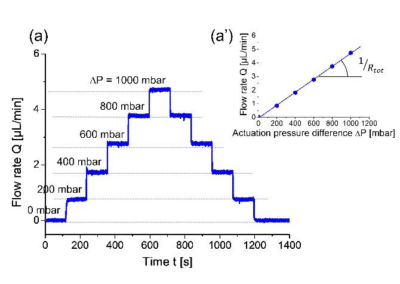
Scanning transmission soft X-ray spectromicroscopy in water
The authors also demonstrated proof-of-principle observations by performing in-situ observations of calcium carbonate nanoparticles. A typical image of mineral precipitate in aqueous medium, acquired at the Ca L2-edge, is shown in Fig. 4a. XAS measurements were performed to confirm that calcium is present within the particles and almost undetectable in the surrounding liquid (Fig. 4b). X-ray absorption spectra of the precipitate were recorded, allowing us to specify the amorphous state of the probed material by analyzing the peak positions (Fig. 4c).
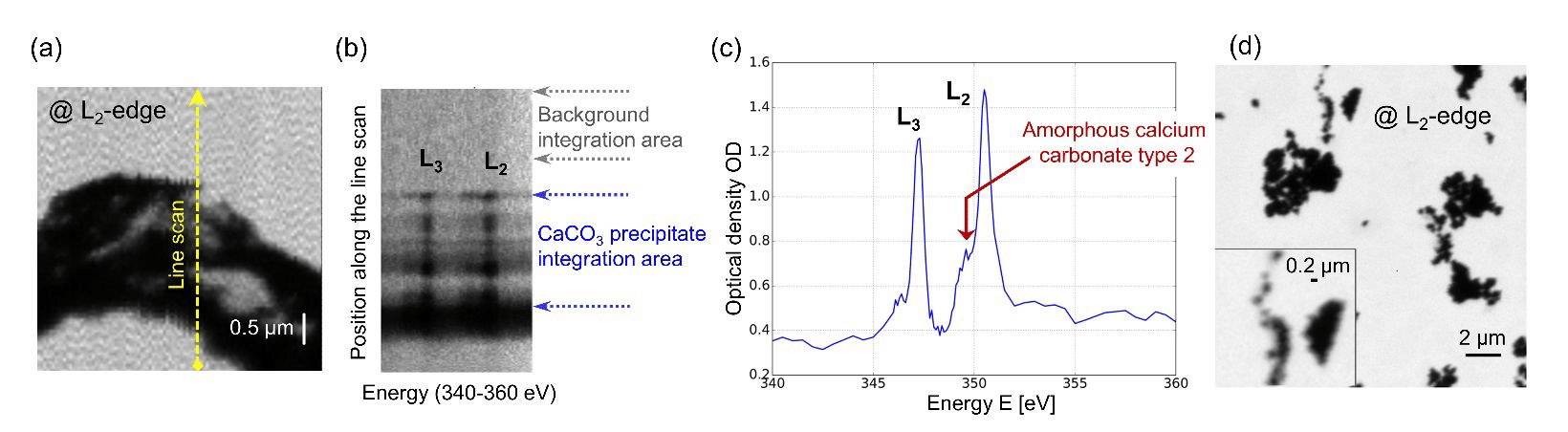