Addressing Air Bubble Issues in Microfluidic Systems
Air bubbles, a common issue in microfluidic experiments, can significantly affect performance and reliability of the setups, impacting experimental outcomes and data accuracy. Addressing this issue is crucial for advancing the field.
Issues created by air bubbles
Microfluidics have revolutionized various fields including biology, chemistry, and medicine.[1] However, one of the common challenges faced in microscale fluidic experiments is the presence of air bubbles. In such intricate systems, simple taping of the finger to the device would not work. Their presence can significantly affect the performance and reliability of microfluidic devices, leading to inaccurate results or even device failure. This expertise page provides a comprehensive understanding of bubble-related challenges faced by researchers, offering insights into their sources, negative effects on experiments, and effective strategies to prevent and eliminate them from microfluidic setups.
Where do air bubbles come from?
Bubbles can vary in size from a microscopical to a visible scale. In the context of microfluidic systems, they are typically small due to the microscale dimensions of the devices, but their impact can be significant. Gas bubbles can originate from various sources. Understanding their formation process is the first step towards developing strategies to prevent them from forming and improve the performance and reliability of the experiments.
Dissolved Gases
One common origin of air bubble formation is the presence of dissolved gases in the liquid. Microfluidic experiments usually involve the movement of liquids that can create pressure differentials. High-pressure conditions within microchannels can contain significant amounts of dissolved gases. When pressure decreases, gas solubility diminishes, making the formation of bubbles more likely. Temperature changes can also influence gas solubility(figure1). For instance, when a liquid reagent is introduced directly from a refrigerated environment, it enters the system at a lower temperature than the fluids in the system, increasing the potential for bubble apparition.
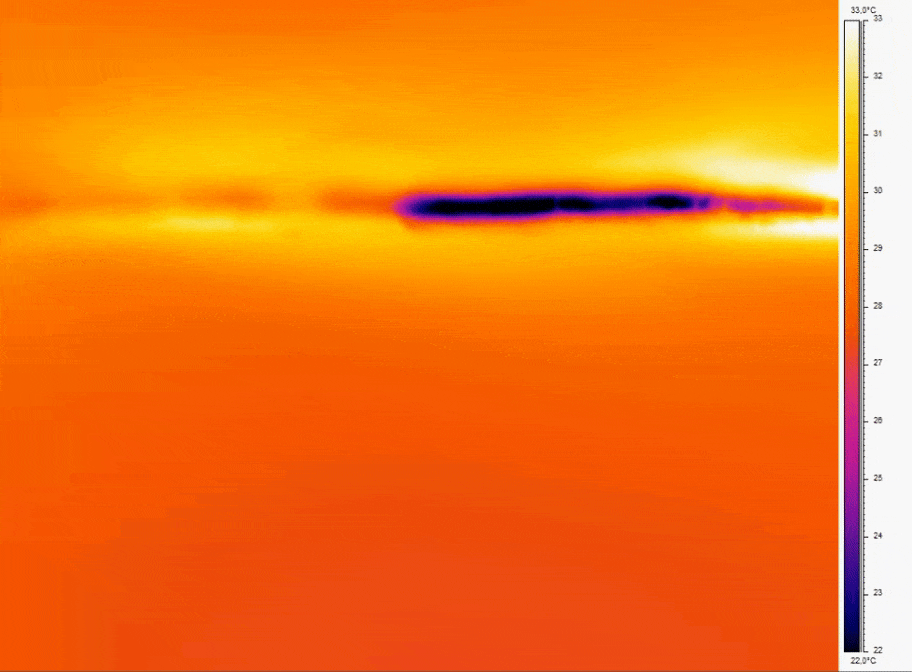
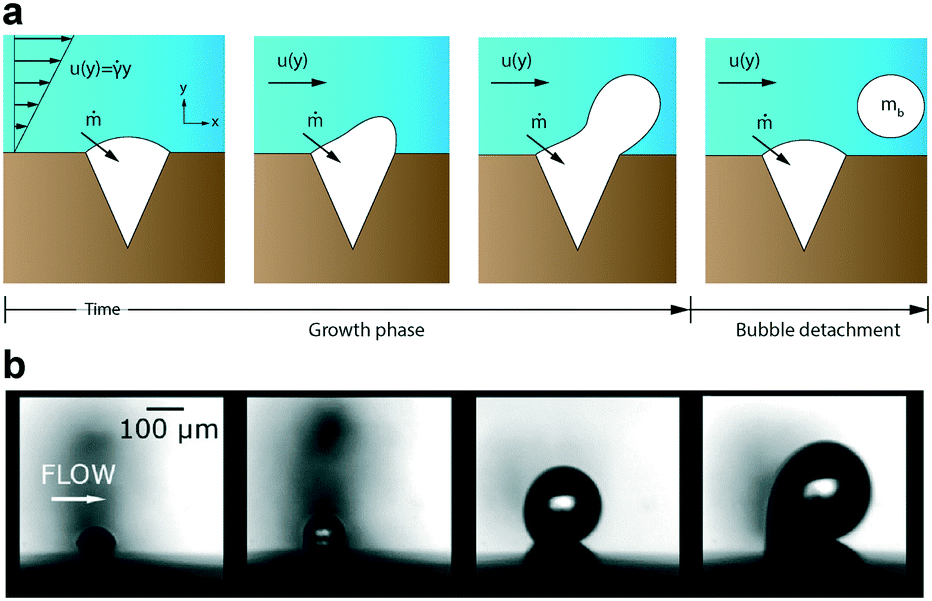
Bubbles do not spontaneously emerge due to environmental changes alone. Minuscule irregularities found on the surfaces within the microfluidic system act as sites where tiny volumes of air can become trapped(figure2a). Under stable conditions, the trapped gas remains static. Yet, any perturbation in the surrounding liquid’s pressure can either dissolve the gas or induce a gas flux within the irregularity, causing the air pocket to grow(figure2b). It’s important to note that the nucleation point remains in the same location, giving rise to the possibility of new gas bubbles forming in the future.[2]
Physical characteristics of microfluidic setup
The configuration of microfluidic platform also plays a role. Hydrophobic surfaces and surface roughness on channel walls can trap air pockets, creating sites for bubble formation. Some components within microfluidic devices, such as valves and pumps, may act as bubble generators during their operation. Abrupt changes in channel geometry such as sharp angles, corners, sudden expansions or contractions, can also induce pressure fluctuations, leading to generation of bubbles (figure 3).
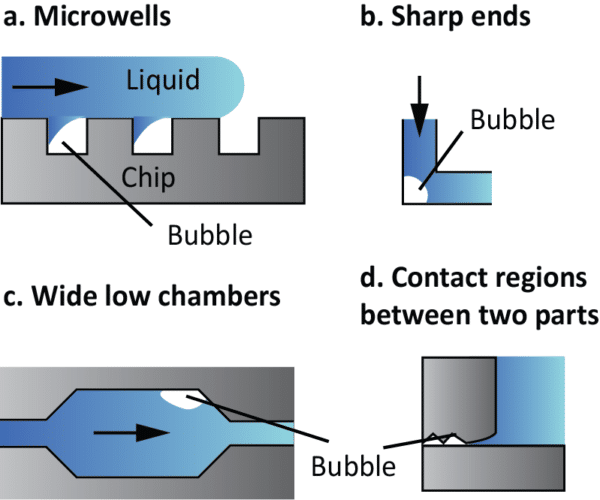
Porous material
The introduction of air into your system doesn’t only occur during the filling step of channels or when there are leaks that allow air to enter. In fact, microfluidic components are often made from materials like polydimethylsiloxane (PDMS), which are permeable to gases. Ambient air can pass through the device walls, leading to the gradual formation and accumulation of small bubbles within the liquid channels.
Chemical Reactions and Fluid properties
Some chemical reactions used in microfluidic experiments can produce gases as a byproduct, resulting in the release of gases in the solution. Even the presence of surface-active agents, like surfactants, can either promote or inhibit formation of bubbles, depending on their concentration and properties.
Troubles caused by bubbles
Flow Instability
The presence of bubbles can disrupt the uniformity and consistency of fluid movement through microchannels, causing an important flow instability. This can lead to unwanted fluctuations in flow rate and pressure. Flow instability can compromise the reliability and reproducibility of experiments, affecting the accuracy and success of microfluidic processes.
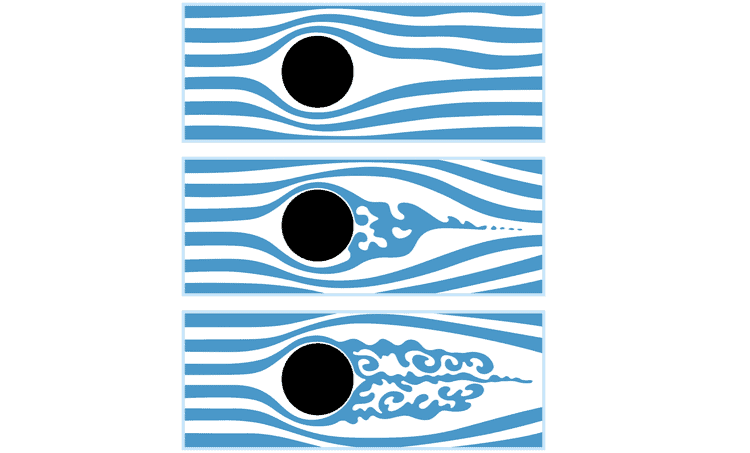
System Response Time
Bubbles can significantly affect the response time of microfluidic systems. When they enter the microchannels, they can create delays in system response. This is especially relevant in applications that require rapid changes in fluid composition, concentration, or flow rates. As a result, the overall efficiency and real-time control of microfluidic processes may be compromised.
Clogging of Microfluidic Networks
The accumulation of bubbles in the setup can lead to physical blockages (figure 5). They become trapped in narrow channels, junctions, or valve openings, preventing the fluid from flowing through the device. This clogging prevents the system from functioning as designed, increases the time needed to reach pressure equilibration, and leads to the failure of many biological and chemical experiments. Clogging does not only prevent the progression of experiments but also necessitates time-consuming maintenance to clear obstructions.
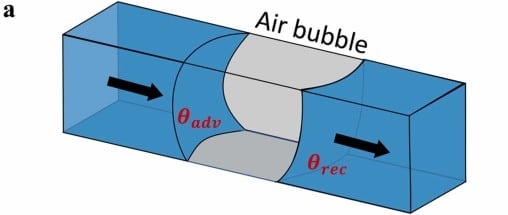
Damage to Cell Membranes
In biological applications, air bubbles can damage cell membranes, affecting the viability and function of the cells. They present interfacial tension that can apply sheer stress on cells and even lead to cellular death (figure 6). Additionally, the interfaces between the gas bubbles and the liquid are an area where particles and proteins can aggregate, leading to artifacts in the experiment. These artifacts can interfere with the measurement and analysis of the biological samples, reducing the accuracy and reliability of the results.
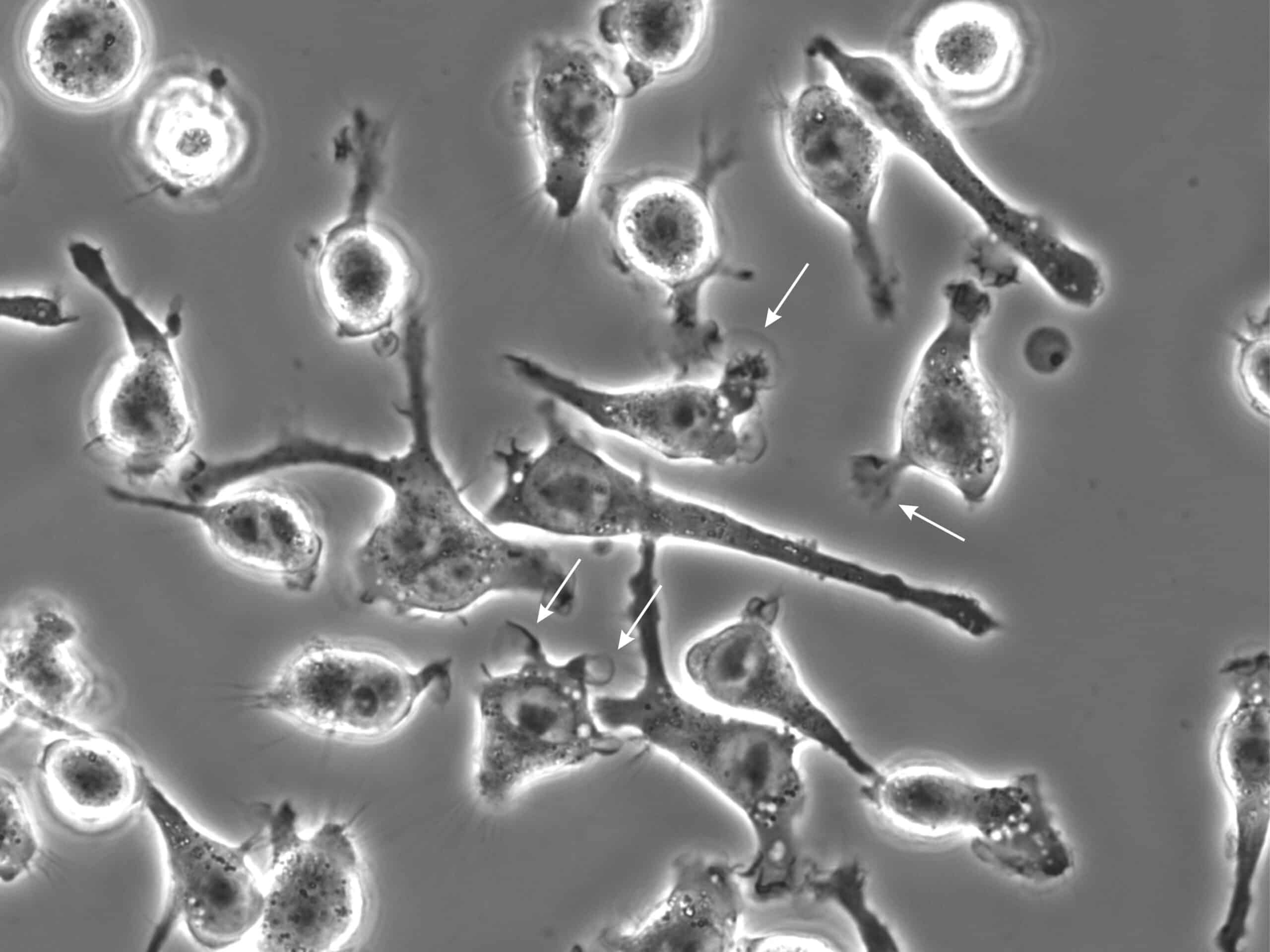
Experimental Interactions and Issues/Analytical Interferences
Air bubbles can alter chemical mixing by acting as physical barriers. These barriers prevent the chemicals from properly touching each other. As a result, the speed of reactions and the outcome are affected.
These tiny air pockets can also distort detection outcomes, causing inaccurate readings. They can obstruct current conduction and alter optical paths, impacting measurements and observations.
This can cause incorrect measurements of light absorption or fluorescence, leading to inaccurate data collection and interpretation. Furthermore, bubbles passing through microfluidic channels can damage the functionalization of the microfluidic wall, such as removing previously made chemical grafting.
How to avoid air bubbles in microfluidics setup
Design optimization
Optimize the design of microfluidic setups to minimize geometries that increase the probability of generating bubbles. Smooth transitions between different channel widths and shapes can reduce pressure fluctuations that trigger their formation. Additionally, ensuring correct installation and configuration of all system components can prevent air from entering the system.
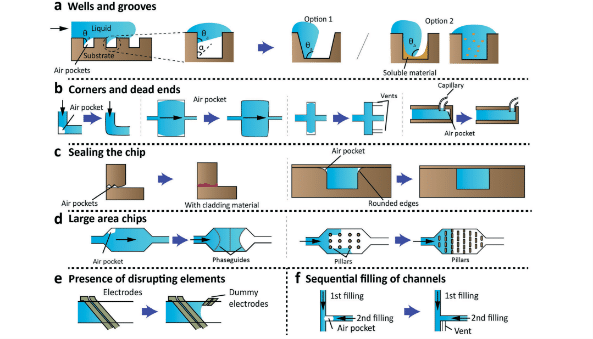
Material Choice
To limit gas diffusion, choose materials with low gas permeability designed for microfluidic applications. To decrease the probability of bubble entrapment, opt for hydrophilic surfaces and apply treatments that enhance this property.
Flow Control
Implementing a flow controller, such as the Flow EZTM, into your setup ensures that pressure variations are minimal, reducing the risk of air bubble formation. By monitoring and adjusting pressure flow rate in microfluidic applications such as droplet generation, users improve the overall reliability of the experiment compared to other methods like syringes and peristaltic pumps.
Acting on bubbles
Active degassing
There are different methods for degassing liquids, such as vacuum degassing, helium sparging or sonication[4]. However, these methods have some limitations, such as high cost, high maintenance, high noise, high waste, and/or low efficiency. A new solution that overcomes these limitations is using a Degasser. This device prevents the formation of unwanted bubbles and also removes already formed bubbles. It uses an innovative non-porous semi-permeable membrane that allows gas molecules to diffuse out of the liquid and into a vacuum chamber.
Passive bubble removing
Incorporate a Bubble trap to eliminate unwanted bubbles that are already traveling with the microfluidic system. It can be integrated into the microchannel design and is particularly effective for preventing them from reaching sensitive regions of the setup (such as microfluidic chips). Thanks to a hydrophobic micro-porous gas-permeable membrane (PFET) this trap is designed to capture and isolate visible bubbles from le water-based flow that may form during the experiment. When the fluid containing gas bubbles flows through the trap, they are expelled through the hydrophobic membrane that allows no aqueous liquid to leak.
Conclusion
On this page, we focused on the importance of addressing air bubbles in microfluidic systems. They can originate from various sources, potentially disrupting experiments and compromising data accuracy. To resolve this issue, we recommend optimizing system design, material selection, and flow control. Additionally, using degassing methods and incorporating Bubble traps in the microfluidic setup can effectively prevent and remove bubbles. In essence, a combination of these strategies is essential to ensure reliable and accurate experiments.
Related products
Related Resources
- Expert Reviews: Basics of Microfluidics
How to choose a microfluidic chip
Read more - Expert Reviews: Basics of Microfluidics
Microfluidic Flow Control: Comparison between peristaltic, syringe and pressure pumps for microfluidic applications
Read more - Array
Microfluidic Flow Control Technologies: Strengths and Weaknesses
Read more - Expert Reviews: Basics of Microfluidics
Microfluidic volume definitions
Read more - Microfluidic Application Notes
Microbubble formation using the RayDrop
Read more
References
- [1] Lab-on-a-Chip: A Revolution in Biological and Medical Sciences., Analytical Chemistry 2000 72 (9), 330 A-335 A, DOI: 10.1021/ac002800y
- [2] Pereiro, Iago; Fomitcheva Khartchenko, Anna; Petrini, Lorenzo; Kaigala, Govind V. (2019). Nip the bubble in the bud: a guide to avoid gas nucleation in microfluidics. Lab on a Chip,
- [3] Zhao X, Ma C, Park DS, Soper SA, Murphy MC. Air bubble removal: Wettability contrast enabled microfluidic interconnects. Sens Actuators B Chem. 2022 Jun 15;361:131687. doi:10.1016/j.snb.2022.131687. Epub 2022 Mar 12. PMID: 35611132; PMCID: PMC9124586.
- [4] Int J Pharma Res Health Sci. 2020; 8 (4): 3195-202 3195; DOI:10.21276/ijprhs.2020.04.02; K Raval and H Patel