Agarose Microcapsules Synthesis
Microfluidic techniques show great power for generation of highly monodisperse multiple emulsion droplets with structural diversity. This can allow for the design and controlled generation of microcapsules with well-controlled sizes, shapes, compositions, and structures as well as integrated functions for controlled drug release [4].
The production of agarose microcapsules is of increasing interest due to the numerous advantages of agarose. Agarose-based polymers are naturally inert and are characterised as biocompatible materials that are well suited for use as carriers for various biomedical and biotechnological applications. [11, 12].
Following is a double emulsion generation method to obtain agarose microcapsules with total control of microcapsule formation and without the drawbacks of large size distribution that present in other methods. The method is performed with the RayDrop (developed and manufactured by Secoya).
Secoya developed and manufactured the RayDrop used to perform this application note.
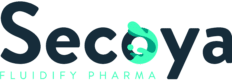
Introduction
Microcapsules are becoming commonly used drug delivery systems as they can be easily administered and can be engineered with different structures and functions for keeping drug stability, delivering drugs to a desired location, and releasing drugs at a predetermined rate in a well-controlled manner [3].
Droplet-based microfluidics has been used as a tool for small scale single-cell or cell culture analysis, chemical synthesis as well as high-throughput screening [4]. Achieved by dispersions of stabilized liquids within continuous immiscible fluids, thousands of particles are generated within minutes. These can be used as microcompartments suitable for such experiments [6]. Gaining in usage are water-in-oil-in-water (w/o/w) and oil-in-water-in-oil (o/w/o) emulsions, which can be employed for applications such as drug delivery vehicles, cell carriers, barcoding of droplets, microscale sensors, and more [5].
For these applications, the use of agarose microcapsules is expanding due to the advantages of this polymer. Agarose is a natural polysaccharide obtained from red seaweed and in aqueous solution can form a hydro-gel at low temperatures.
Agarose microcapsules are a well-established for a variety of applications such as protein detection, DNA hybridization and as a mean to immobilize biomolecules in order to support reactions and to permit a faster molecular detection with higher sensitivity and lower reagent consumption. This makes them an ideal choice for microscaled lab-on-chip devices. More specifically, microcapsule-based microfluidic platforms enhance planned chemical or biochemical reactions, increasing the degree of interaction between the biomolecules and the functionalized surfaces [15].
Agarose microcapsules production: Materials and methods
Materials: Reagents
Producing a double emulsion requires 3 phases: the continuous phase, shell phase and core phase. The shell phase must be immiscible with both other phases.
Continuous phase:
- MCT 2: MCT with 2% w/w PGPR (E576)
Shell phase:
- Ag1: MiliQ water with 1% w/w TWEEN 80 and 1%w/w Agarose
- Ag2: MiliQ water with 1% w/w TWEEN 80 and 2%w/w Agarose
- Ag3: MiliQ water with 1% w/w TWEEN 80 and 3%w/w Agarose
Core phase:
- MCT 1: MCT oil only
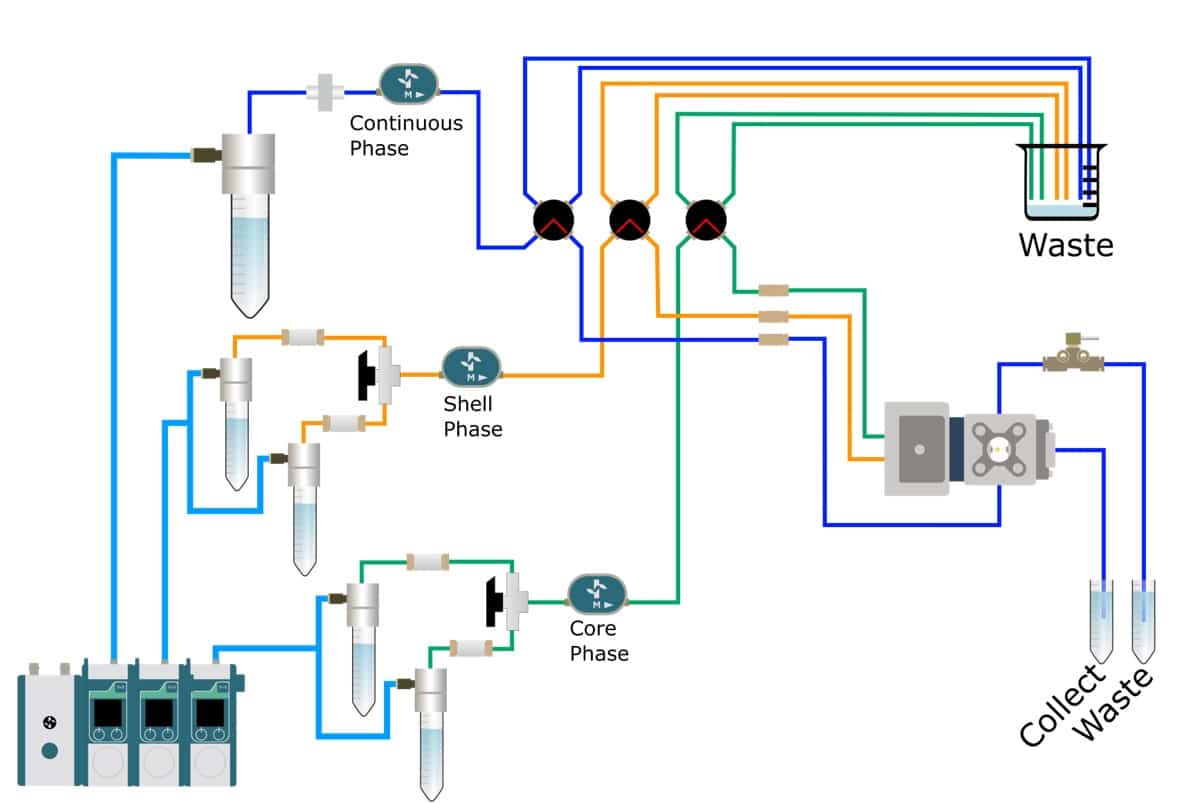
Materials: Products
Double emulsion production method: How to synthetize agarose microcapsules?
Oil-core agarose shell emulsions are manufactured using a RayDrop 90-160-450 due to its flexibility and stability.
Agarose microcapsules production is performed in several steps:
- Preparation:
It’s recommended to filter all liquids to avoid clogging and to degas solutions to minimize the apparition of air bubbles inside the system.
All fluidic lines of the setup have to be sequentially filled with liquid (continuous phase, core phase and shell phase) to prime the system and ensure that it’s wet and free of air bubbles.
- Shell Phase Simple Emulsion:
Production of a single emulsion of the shell phase in the continuous phase, increasing the pressure until reaching a jetting mode.
- Double Emulsion:
Production of a double emulsion adjusting the core flow, due to the shearing of this phase with the previous phase (single emulsion droplet already formed).
- Droplet collection:
After gelation is complete, the microcapsules are collected on a filter/sieve, washed with excess water and resuspended in MCT oil working solution.
- Production run:
Oil core – hydrogel shell microcapsules are produced at gram quantities. The system was left running for at least 30 minutes to determine the long-term stability and ability to withstand clogging.
Production of Agarose microcapsules: RESULTS
Ag1 (1% agarose solution) and Ag2 (2% agarose solution) have resulted in the successful formation of oil core–agarose shell microcapsules.
Ag3 (3% agarose solutions) did not result in stable droplet formation due to the to the high viscosity of the solution.
Premium quality agarose microcapsules were successfully obtained. Droplet formation was stable enough to enable long-term production of the oil core – agarose shell sample over the course of 180 minutes.
For a configuration with the nozzle and output capillaries (respectively 90µm and 450µm as presented in this note), adjusting the flow rates of the fluids allows for fine control of the capsule dimensions (see Figure 2). With this setup, agarose microcapsules from 215µm to 300µm are easily produced. The shell thickness of microcapsules can also be varied by changing the ratio of flow rates of the shell and core phases, as shown in Figure 3. Here core thickness varies from 80µm to 170µm.
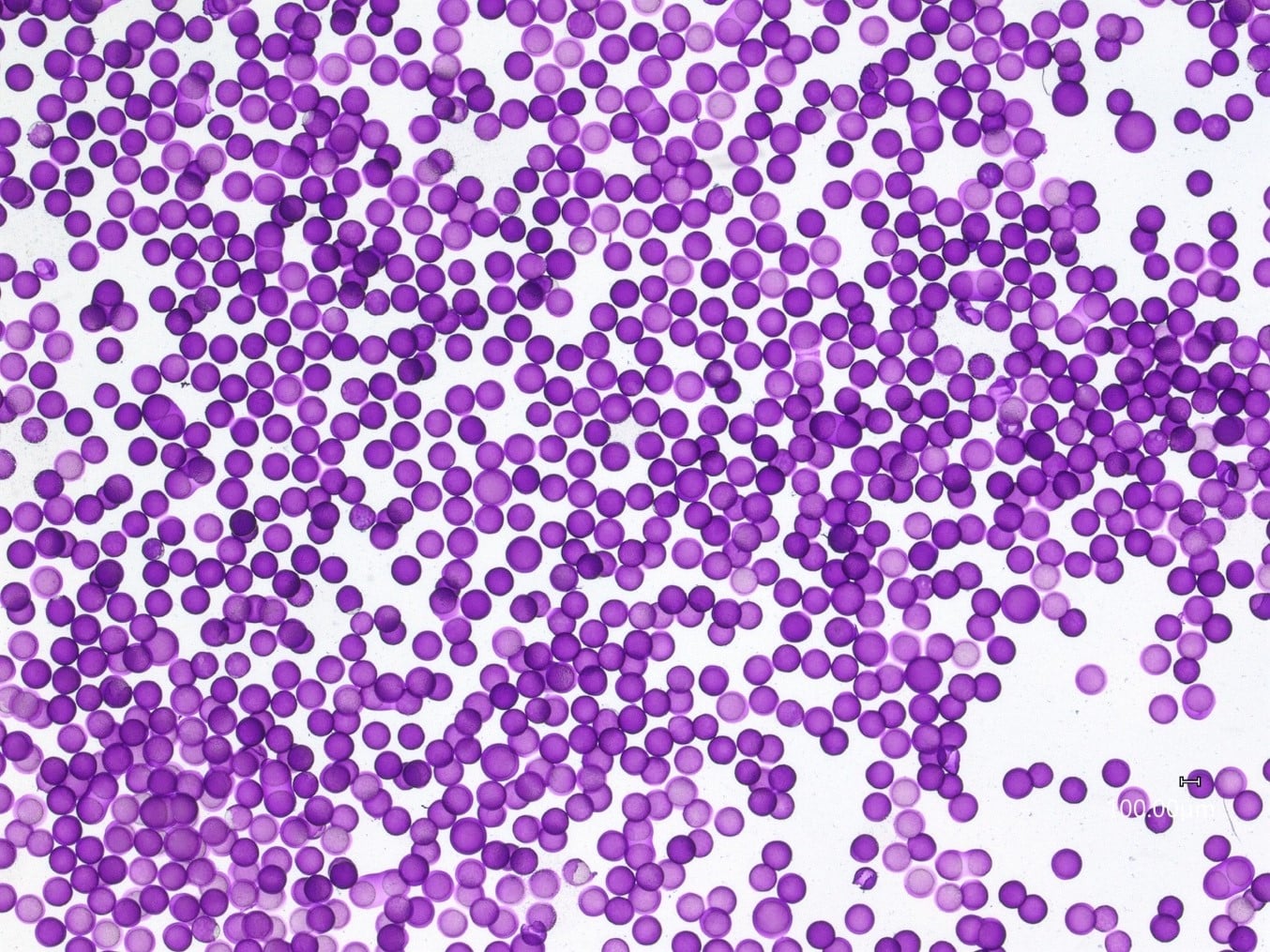
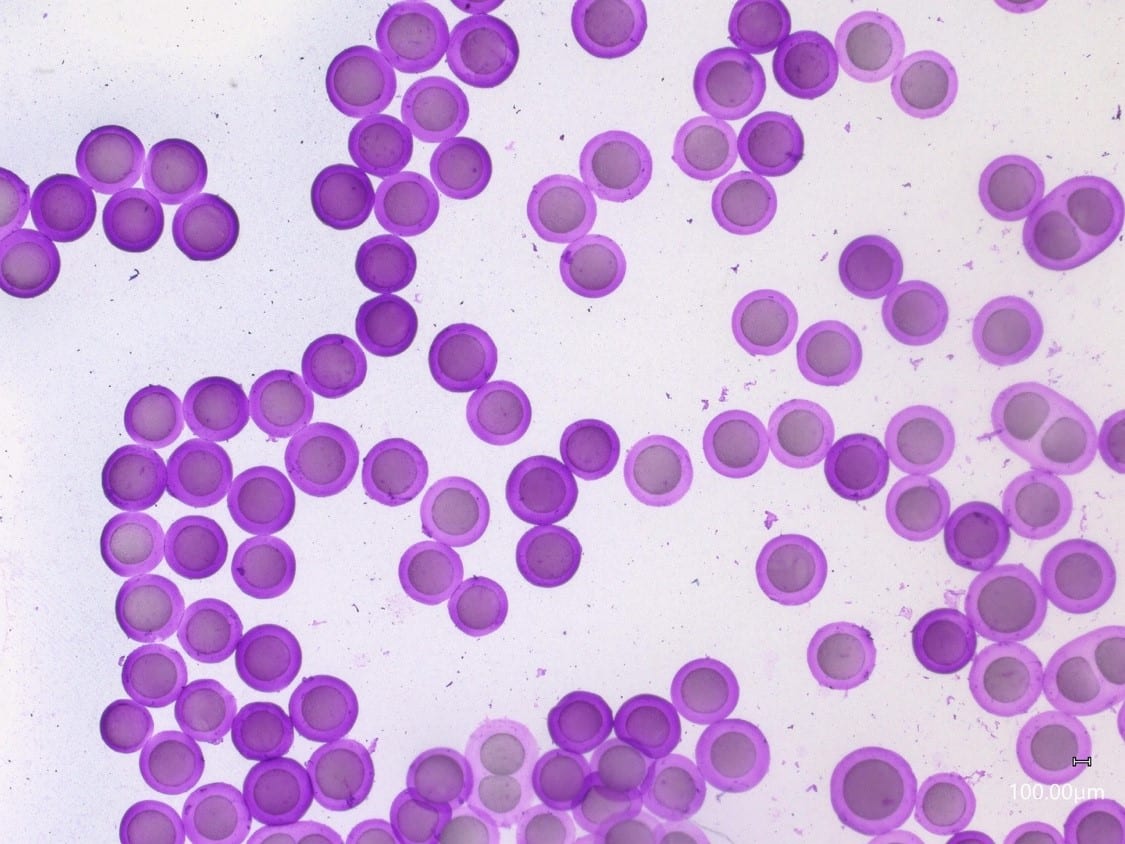
Conclusion
Using Fluigent’s double emulsion production station it is possible to generate agarose microcapsules over the outside diameter range of 215 and 300 µm with standard RayDrop configuration (Nozzle of 90µm and outlet capillary 150µm) using agarose solution at 1% in water. Other concentrations of agarose such as 2%, which is widely used in biological applications, have also been successfully tested by following the same process.
This demonstrates that the RayDrop double emulsion and Fluigent liquid control technology can be successfully used together to produce double emulsions. The ability to produce highly monodisperse agarose microcapsules has also been demonstrated.
Variation of the continuous phase flow does not produce a significant change in droplet formation rate or size – this further adds to the stability of the system. Long-term production of agarose microcapsules (over 180 minutes) was successfully undertaken.
The droplet size variation can be successfully controlled by altering the flow rates of the droplet phases and/or the size of the Raydrop chips. This also shows that core-shell ratio can be successfully adjusted by altering the relative flow rates of core and shell phases.
This confirms that the Raydrop™, in combination with Fluigent pumping technology, are one of the most competitive double emulsion systems available due to the flexibility (changing capillary size can be easily done to target different droplet sizes), the ease of use, the breadth of accessible chemical systems, the long-term performance stability and the lack of coatings (e.g. sigma coat)
References
1. Bae, Y., & Park, K. (2011). Targeted drug delivery to tumors: Myths, reality and possibility. Journal Of Controlled Release, 153(3), 198-205. doi: 10.1016/j.jconrel.2011.06.001
2. Delcea, M., Möhwald, H., & Skirtach, A. (2011). Stimuli-responsive LbL capsules and nanoshells for drug delivery. Advanced Drug Delivery Reviews, 63(9), 730-747. doi: 10.1016/j.addr.2011.03.010.
3. Ma, Z., Li, B., Peng, J., & Gao, D. (2022). Recent Development of Drug Delivery Systems through Microfluidics: From Synthesis to Evaluation. Pharmaceutics, 14(2), 434. doi: 10.3390/pharmaceutics14020434U.
4. Khan, I., Serra, C., Anton, N., & Vandamme, T. (2014). Production of nanoparticle drug delivery systems with microfluidics tools. Expert Opinion On Drug Delivery, 12(4), 547-562. doi: 10.1517/17425247.2015.974547
5. He, F., Zhang, M., Wang, W., Cai, Q., Su, Y., & Liu, Z. et al. (2019). Designable Polymeric Microparticles from Droplet Microfluidics for Controlled Drug Release. Advanced Materials Technologies, 4(6), 1800687. doi: 10.1002/admt.201800687
6. De La Vega, J., Elischer, P., Schneider, T., & Häfeli, U. (2013). Uniform polymer microspheres: monodispersity criteria, methods of formation and applications. Nanomedicine, 8(2), 265-285. doi: 10.2217/nnm.12.210.
7. Guo, J., Hou, L., Hou, J., Yu, J., & Hu, Q. (2020). Generation of Ultra-Thin-Shell Microcapsules Using Osmolarity-Controlled Swelling Method. Micromachines, 11(4), 444. doi: 10.3390/mi11040444
8. Mou, C., Wang, W., Li, Z., Ju, X., Xie, R., & Deng, N. et al. (2018). Trojan-Horse-Like Stimuli-Responsive Microcapsules. Advanced Science, 5(6), 1700960. doi: 10.1002/advs.201700960
9. Zhang, M., Zhang, P., Qiu, L., Chen, T., Wang, W., & Chu, L. (2020). Controllable microfluidic fabrication of microstructured functional materials. Biomicrofluidics, 14(6), 061501. doi: 10.1063/5.0027907
10. Chong, D., Liu, X., Ma, H., Huang, G., Han, Y., & Cui, X. et al. (2015). Advances in fabricating double-emulsion droplets and their biomedical applications. Microfluidics And Nanofluidics, 19(5), 1071-1090. doi: 10.1007/s10404-015-1635-8
11. Kim, S., Kim, J., Cho, J., & Weitz, D. (2011). Double-emulsion drops with ultra-thin shells for capsule templates. Lab Chip, 11(18), 3162-3166. doi: 10.1039/c1lc20434c
12. Vurchio, F., Ursi, P., Buzzin, A., Veroli, A., Scorza, A., & Verotti, M. et al. (2019). Grasping and Releasing Agarose micro-Beads in Water Drops. Micromachines, 10(7), 436. doi: 10.3390/mi10070436
13. Thompson, J., & Bau, H. (2010). Microfluidic, bead-based assay: Theory and experiments. Journal Of Chromatography B, 878(2), 228-236. doi: 10.1016/j.jchromb.2009.08.050
14. Pinto, I., Caneira, C., Soares, R., Madaboosi, N., Aires-Barros, M., & Conde, J. et al. (2017). The application of microbeads to microfluidic systems for enhanced detection and purification of biomolecules. Methods, 116, 112-124. doi: 10.1016/j.ymeth.2016.12.005
15. Du, N., Chou, J., Kulla, E., Floriano, P., Christodoulides, N., & McDevitt, J. (2011). A disposable bio-nano-chip using agarose beads for high performance immunoassays. Biosensors And Bioelectronics, 28(1), 251-256. doi: 10.1016/j.bios.2011.07.027